Hyperbaric oxygen
The basis of hyperbaric oxygen (HBO) treatment are the relationships for inert gases between pressure and volume, and pressure and dissolution in a liquid: i) the pressure of an inert gas is inversely proportional to its volume (Boyle’s law; named after R.W. Boyle, 1627-1691); ii) the amount of dissolved inert gas in a liquid is directly proportional to the partial pressure of the gas in contact with the liquid (Henry’s law; W. Henry, 1803). Henry’s law is illustrated in Figure 1.
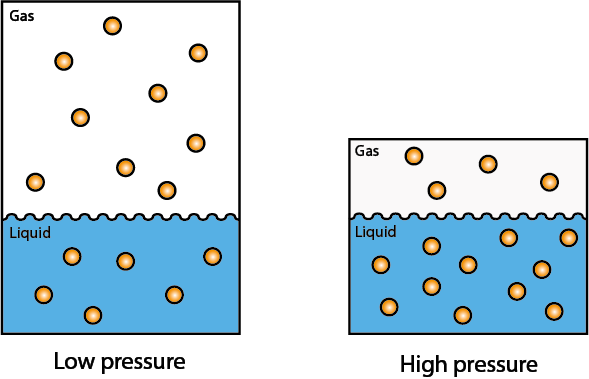
Both Boyle’s and Henry’s laws are approximations, valid only for an ideal gas. But both laws do account for the general trends observed in real gases and liquids.
A rather pleasant applied example of Henry’s law is champagne (or any other bubbly drink). Champagne is bottled under elevated carbon dioxide, CO2, pressure and the amount of CO2 dissolved in the water / alcohol mixture is thus higher than at normal ambient conditions. Upon opening the champagne bottle (decompression) CO2 molecules (in little bubbles) will start to evaporate from the liquid until the appropriate, smaller amount of CO2 remains dissolved in the liquid according to normal ambient pressure (at which point the champagne will have lost its sparkle…).
The normal atmosphere around us is roughly made up of ca. 80 percent nitrogen, N2, and ca. 20 percent oxygen, O2, molecules (and a few, rare noble gas molecules) and the ambient pressure is taken as the ‘normal’ pressure of 1 atm. That is what we normally breathe and what our bodies are used to process through our blood and lungs. Keeping Henry’s and Boyle’s laws in mind, we can explain what can happen to a scuba diver from resurfacing from a deep dive too rapidly. At deep-dive conditions, the diver’s body will have been in an environment of elevated pressure, and more than the normal amount of O2 and N2 molecules will dissolve in their body fluids. As a result of insufficient decompression on resurfacing, mostly N2 gas bubbles will escape into body tissues where they will cause local damage and/or will block the regular blood flow to small peripheral blood vessels. This is known as decompression sickness. The solution to the problem is recompression with breathing elevated O2 levels (HBO) at about three times the normal oxygen partial pressure (followed by controlled decompression).
A slightly different mechanism explains why HBO is an effective antidote to CO poisoning. The CO molecule has a greater affinity to the molecules in our blood that normally carry around O2 molecules (hemoglobin). That means that O2 molecules at normal partial gas pressure will not be able to competitively eliminate the poisonous CO molecules from the body and the body will die from internal suffocation because the hemoglobin molecules cannot deliver O2 molecules to body tissues. In order for the O2 molecules to outcompete the CO molecules, much more O2 and at higher pressures than normal must be applied – HBO, again about three times the normal oxygen partial pressure, does the trick.
Of course, these basic principles of gas exchanges between different kinds of gases and between gas and liquid (or semi-liquid) phases are still in operation when HBO is used as a therapy to fight some severe infections or to treat and/or prevent tissue necroses. In our rather simple examples above a roughly three-fold increase of partial oxygen pressure was mentioned. That is also the level of increased oxygen pressure used in these therapeutic sessions. The increased dissolution of O2 molecules during HBO sessions reverts to normal levels of concentrations in body fluids after decompression.
The therapeutic scenario when treating infections or bone necrosis with HBO is far more complicated than in our illustrative examples. Now a whole range of complicated biochemical and physiological relationships needs to be taken into account, none of which can be properly explained or understood in such simple terms and there will be no simple cause & effect relationships.
First of all, the transport mechanism of O2 around the body under HBO conditions is different from normal conditions. The hemoglobin molecules that normally carry O2 in arterial blood are more or less saturated by O2 molecules under normal pressure and O2 concentration conditions (as in normal air). Under HBO conditions, the additional O2 content is transported by the blood plasma. The biochemical and physiological implications of this modified transport mechanism are not known. Second, O2 is a highly reactive chemical, an oxidizing agent with multiple roles in a cell’s metabolism. It is not well researched and not well understood exactly what effects increased O2 levels have on the cellular metabolism and life cycle. It is a suggestion that increased O2 levels may be helpful because infected and necrotic tissues tend to have insufficient blood supply and thus tend to be hypoxic. This hypothesis has not been fully verified. What is known and reasonably well understood is the role of O2 in general in triggering and promoting angiogenesis and the synthesis of collagen (both important mechanisms in wound healing). Furthermore, HBO treatments may be problematic in the presence of active malignant disease as it is currently not possible to be certain that elevated O2 levels do not fuel growth of malignant cells.
In addition, there is very little (if any) convincing evidence of the clinical effectiveness of HBO for the prevention and/or treatment of necrosis. The evidence base for the treatment of some severe infections (gangrene) is slightly more convincing. It is likely that the mechanisms by which HBO works for the treatment of (bacterial) infections is different from HBO effects on osteoradionecrosis.
It has been said that ‘HBO is a treatment in search of diseases’ and HBO treatment remains a controversial approach. In the light of lack of evidence (and understanding) there are currently two trials running in the UK, both closing in 2017: the HOPON and DAHNCA 21 trials, investigating the role of HBO in the prevention and treatment of osteoradionecrosis, ORN after radiotherapy in the head and neck region, respectively. For example, the HOPON trial, focusing on learning about the potential of HBO in the prevention of ORN, consistently prescribes a series of 20 HBO sessions before any invasive dental treatment such as a tooth extraction, and after such an intervention another 10 HBO sessions are prescribed. The outcomes of a large group of patients at risk of ORN treated in this way will be compared to the outcomes for a very similar patient group that is looked after and treated in quite the same way as the first group, except that the second group will not have had HBO sessions.