Saliva and oral health
Contents
Composition of saliva
Saliva is a very nearly neutral (that is neither alkaline nor acidic) aqueous solution. Like all body fluids it contains a number of electrolytes (that is inorganic ions) such as Na+, K+, Ca2+ and Mg2+ cations, and Cl-, HCO3-, PO43-, I- and F- anions. Saliva is super-concentrated as far as Ca2+ and PO43- ions are concerned and in this way prevents teeth dissolving in this aqueous electrolyte solution. The electrolytes, in particular HCO3-, act as a buffer (that is they balance and maintain the pH value such that there are no large pH fluctuations).
Organic components of saliva include a large number of proteins. Of the proteins in saliva amylase, statherin and mucins are mostly relevant for the process of chewing, tasting and swallowing but also play a role in maintaining oral health.
Other proteins such as lysozyme or lactoferrin are important for the role of saliva in protecting teeth and oral health in general.
Functional organic components of saliva relevant for oral health
The two most important proteins in saliva for the protection of oral health are lysozyme and lactoferrin. Further proteins in saliva such as mucins, amylase and statherin have their primary role in enabling chewing, eating and swallowing as well as a second role in maintaining oral health.
Lysozyme
Lysozyme is a large protein molecule, it belongs to the class of enzymes (proteins that enable specific chemical reactions in living cells). The role of lysozyme in maintaining oral health is defined by its ability to fight bacteria, lysozyme is a part of our bodies’ defence mechanisms, the immune system. The antibacterial properties of lysozyme were first noted by Alexander Fleming, the discoverer of penicillin. Lysozyme is found in many body fluids such as tears, saliva or mucus to protect body surfaces from bacterial infections. Lysozyme is also found in high concentrations in egg white (to protect the developing chick).
Most bacteria have a cell wall composed of so-called cross-linked peptidoglycan, which gives a stable and difficult to transgress outer ‘skin’ to bacterial cells. This is illustrated in Figure 1.
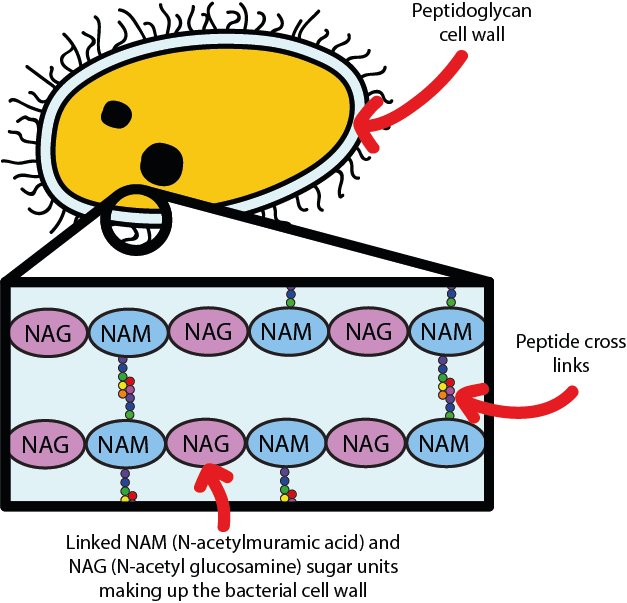
The cell wall protects bacteria from taking up too much water and exploding. Lysozyme kills bacteria by attacking the cell wall, causing it to rupture and the bacterium to die. The mechanism of the attack is shown in Figure 2.
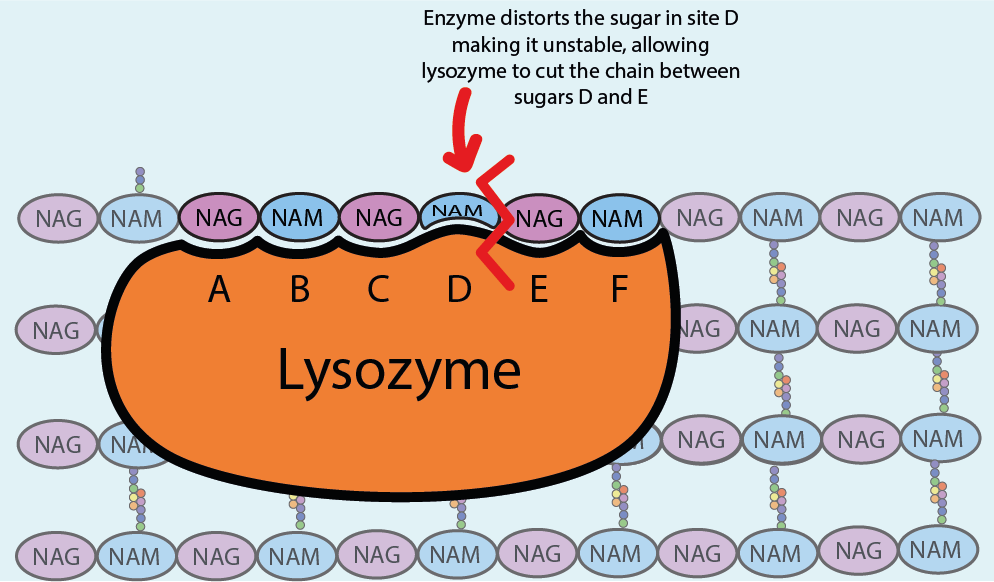
Lysozyme is structured in such a way that its active site is able to wrap around and bind with six of the NAM and NAG sugar units of the cell wall, the peptidoglycan. Upon binding the fit is perfect but the NAM sugar site D in the bacterial cell wall (see Figure 2) is now strained, making it unstable and more reactive. This allows lysozyme to cut the bond between the sugar units in the D and E sites, breaking the peptidoglycan into fragments. Lysozyme then detaches from the site and finds another site to bind with and cut. In this way the bacterial cell wall is increasingly weakened as lysozyme makes more and more cuts in the cell wall.
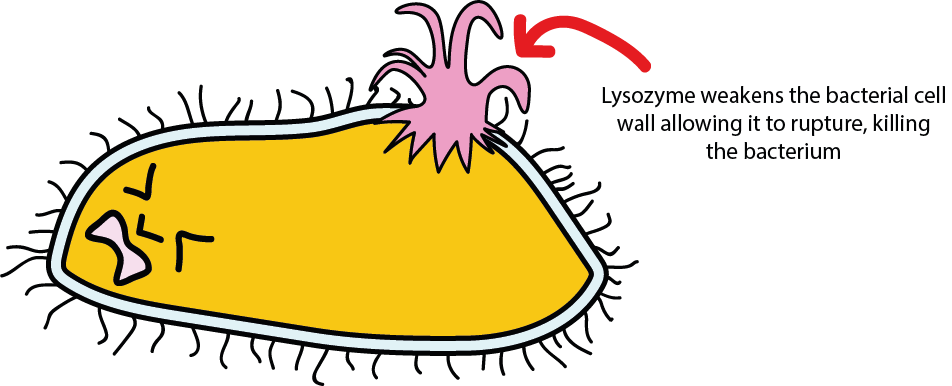
Figure 3 symbolises the final result – once the cell wall is sufficiently weakened, it ruptures. Water then flows into the bacterial cell because the inside of the cell has a high concentration of solutes (dissolved ions and molecules), higher than the concentration in the surrounding tissue. Water continues to flow in until eventually the pressure inside the bacterial cell is such that the cell wall cannot hold the pressure and the bacterial cell literally explodes.
There are no indications that harmful bacteria are going to be able to develop any resistance to this natural antibiotic, lysozyme, any time soon: lysozyme attacks such a fundamental principle of the ‘anatomy’ and functioning of bacteria that they would have great difficulties to adapt their ways to escape from the fatal attack of lysozyme, without dying anyway. Unfortunately the same cannot be said about antibacterial agents invented by humans – these antibiotics are more prone to allow bacteria to learn how to circumvent their actions (that is, become resistant). This is now a widespread publically acknowledged problem.
It may thus appear an obvious solution to use lysozyme in the treatment of bacterial infections. If only it would be that simple! First of all, these kinds of proteins are highly likely to be specific to a particular species (for example, humans will have slightly different versions of them than other animals). Second, think of the very large lysozyme molecule – it is too large to be easily moved around through your digestive system, it cannot be easily delivered to a target site in the body by, for example, using it as a tablet; it has to be made by your body at the location where it is needed.
Lactoferrin
Lactoferrin is a moderately sized glycoprotein molecule (ca. 80 kDa that is found in many body secretions such as human milk, nasal mucus, saliva or tears. It forms part of our body immune system and is known to have antibacterial, antifungal and antiviral properties. The various exact working mechanisms of these multiple actions of lactoferrin are not all known to the same degree of precision as the functioning of lysozyme.
However, several of the many roles and functions of lactoferrin are well characterised. Figure 4 illustrates one of the known mechanisms underlying the antibacterial function of lactoferrin.
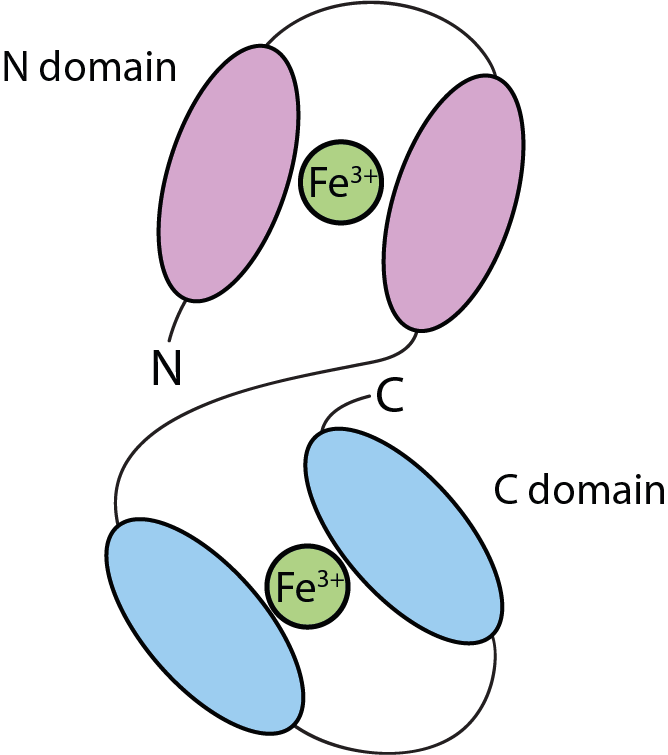
The two main domains in the lactoferrin molecular structure are ideally set up to accommodate Fe3+ ions, thus lactoferrin binding Fe3+ ions from the surroundings makes these ions unavailable to bacteria, and most bacteria need these ions to promote their growth.
Another mechanism of the antibacterial properties of lactoferrin is shown in Figure 5.
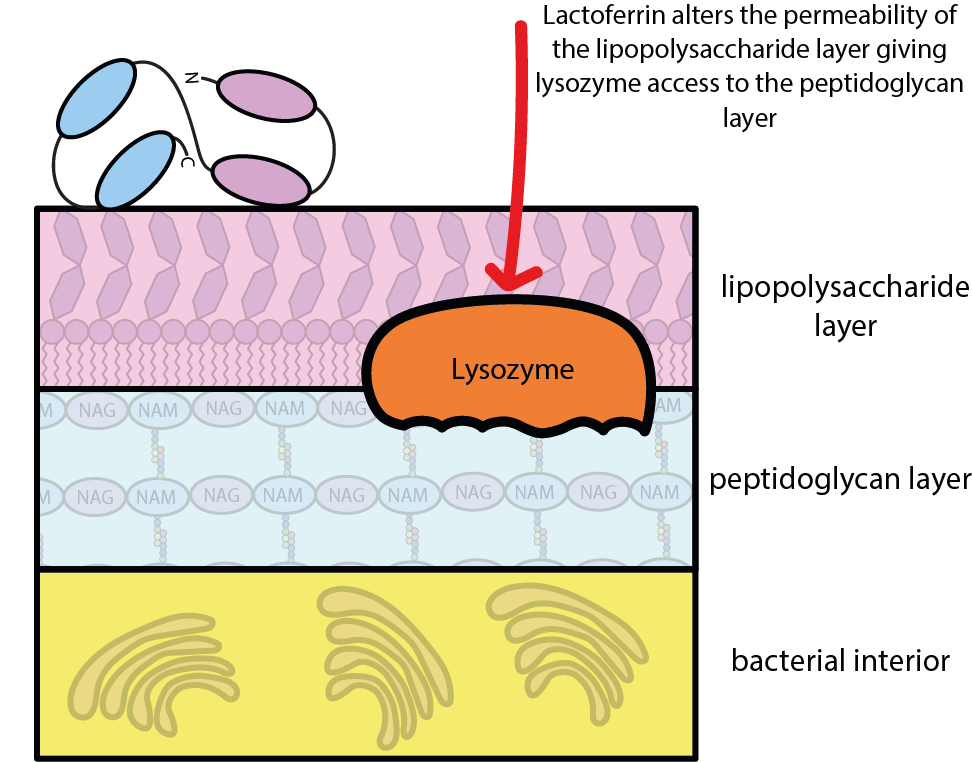
The cell wall of bacteria is protected by a layer of, essentially, fat and sugar molecules (a so-called lipopolysaccharide layer), a little bit in the spirit of impregnating a rain coat. By binding to this protective layer, lactoferrin alters the layer’s permeability and eases the access to the structural part of the cell wall (the peptidoglycan layer) for the lysozyme molecules. Lactoferrin in this way enhances the antibacterial action of lysozyme.
Mucins, amylase and statherin
Mucins are a family of typically very large molecules. Their main role is to give saliva its viscosity, making it sufficiently sticky to not be easily washed away and render it a non-Newtonian fluid (that is a fluid the viscosity of which depends on the shear forces acting on it).
Statherin is an amphiphilic organic / biochemical molecule, a phospholipid with a polar hydrophilic end (hydrophilic means water loving) and a non-polar hydrophobic end (hydrophobic means water hating, sometimes equivalent with fat loving). The polar phosphate end of the molecule attaches itself to the surface (the enamel) of teeth where it helps to protect teeth and to yield a smooth surface of teeth in order to protect tongue and mucosa. This is sometimes termed a “biofilm”.
Alpha-amylase is a large enzyme molecule. Its role as an enzyme is to enable the breaking down of large carbohydrate molecules in food (such as starch in potatoes) into smaller units while chewing and thus help eating. The related role of alpha-amylase in terms of oral health is to help clearing the mouth from debris after eating, including hard to reach spaces such as between teeth. Alpha-amylase is mostly contained in the on-demand saliva made in the parotid gland while eating.