Immunotherapies
The immune system’s role in the defence of the body requires it to recognise and destroy foreign and faulty cells, including malignant cells. In humans (and other mammals) this defence system is a sophisticated mechanism, made up of two interlinked components. The innate immune system provides a rapid, non-specific response; the adaptive immune system uses specialised white blood cells to produce a slower but specific response to invaders, in addition to creating and maintaining a memory of such events and invaders.
The interplay of these different layers of the human immune system is discussed in our section about inflammation, with a focus on infection, wound healing and autoimmune diseases such as Sjögren’s syndrome. In the context of immunotherapies, below we take a look at the immune system from a slightly different angle. The focus here is on the different types of white blood cells (lymphocytes) and their specific properties and roles as these are key for all immunotherapy strategies, aiming to exploit the body’s own defence system to eradicate malignancies.
The idea to counteract malignant diseases by exploiting the body’s own defence mechanisms is as old as the growing understanding of the immune system. The idea has been discussed for at least 100 years but significant clinical progress in applying such strategies in the treatment of some malignancies, in particular some forms of breast, skin and lung cancers has only happened in the last decade.
An overview of white blood cells and other key components in the immune system
The ‘work force’ of the immune system is made up of a number of specialised white blood cells, and a number of additional components to support the overall function and communication flow of the immune system. Figure 1 gives an overview of these key components necessary to provide an adequate immune response.
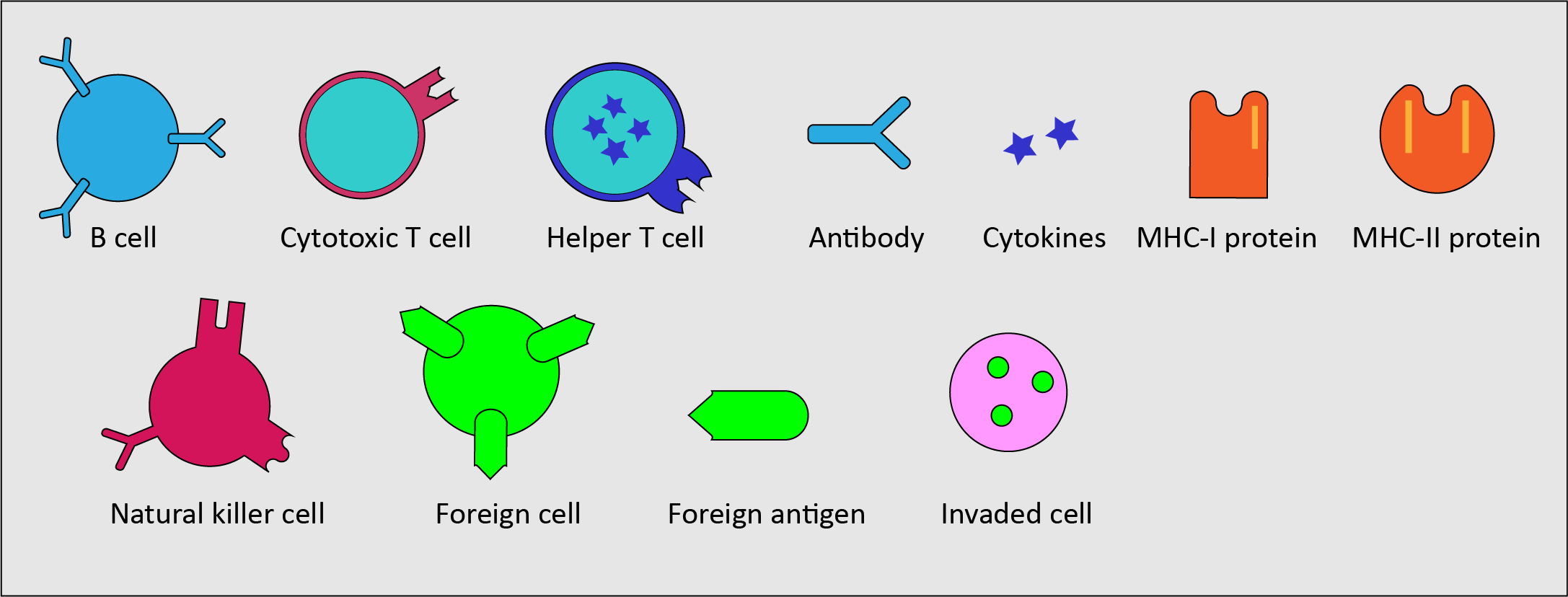
- B cells - a type of white blood cells, a sub-type of lymphocytes; continuously produced in the bone marrow; mostly found circulating in the lymphatic system; part of the adaptive immune system; carry out the synthesis of specific antigens (see below); dealing with invaders of the body fluids outside cells (this is called the humoral immune response).
- T cells - another type of lymphocytes; produced in the thymus (a gland in the chest); mostly found circulating in the lymphatic system; part of the adaptive immune system; identifying foreign invaders (antigens; see below) with the help of specialised receptors (proteins) on the T cell surface; two types of T cells with different properties: cytotoxic T cells (kill infected and malignant cells) and helper T cells (modulate and support the overall immune response).
- Antigens - substances, typically proteins on the surface of pathogens that stimulate an immune response; bind to antibodies and, in modified form, to T cell receptors.
- Antibodies - proteins produced by B cells in response to the presence of antigens; trigger an antigen-specific immune response (each type of antibody recognises a particular antigen); can be found attached to B cells circulating in the bloodstream.
- Cytokines - large variety of small protein molecules, released by various cells of the immune system; act as messenger molecules in regulating the immune response.
- Major histocompatibility complexes (MHC) - proteins expressed by many body cells to label cells as belonging to the body (‘self’); required for (partially broken down) antigens to bind to T cell receptors (said to be ‘presenting’ antigen fragments to the T cell receptors); two types of MHC proteins: MHC-I proteins present to cytotoxic T cells, MHC-II proteins present to helper T cells, followed by the corresponding immune response(s).
- Natural killer cells – a type of lymphocyte, mostly found circulating in the bloodstream; part of the innate immune system; act in similar ways to cytotoxic T cells of the adaptive immune system, but do not require activation and respond rapidly.
Basic steps of the normal immune responses
Natural killer cells recognise infected or malignant host cells or foreign cells by a lack of MHC-I proteins expressed on their surfaces, or by the presence of foreign antigens. Normal healthy cells present enough MHC-I protein to inhibit natural killer cells. If sufficiently stimulated by a lack of MHC-I protein or by presence of foreign antigen, the natural killer cells proceed to kill the identified unwanted cell (Figure 2).
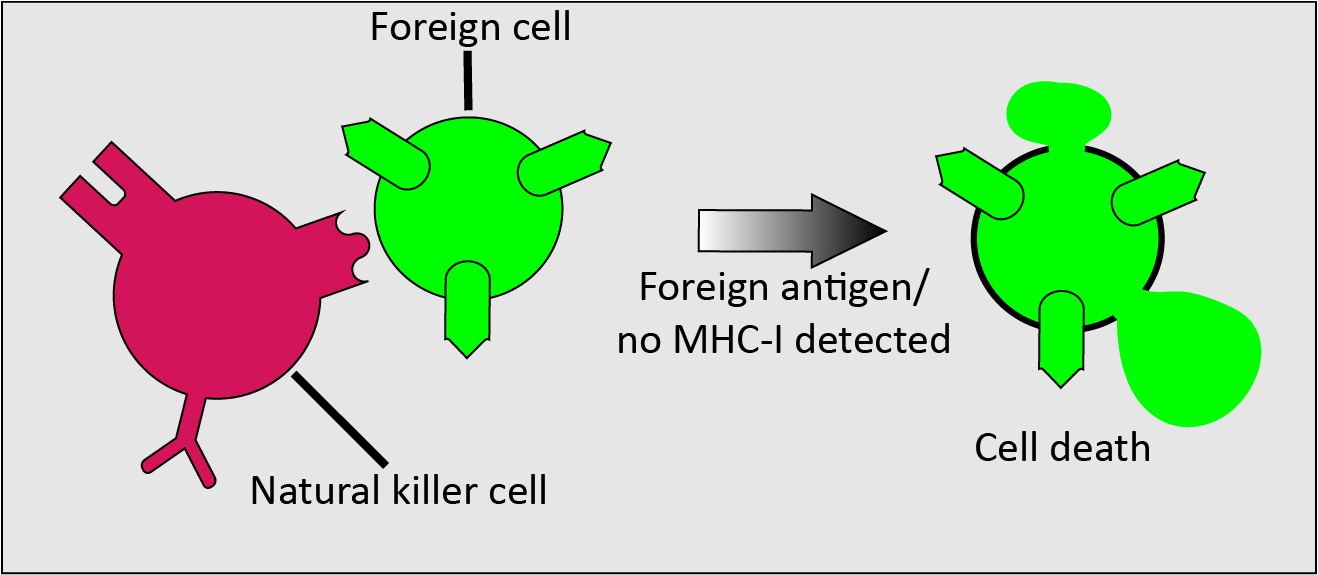
The identification of infected and malignant cells based on reduced levels of MHC-I proteins and the subsequent rapid elimination of such unwanted cells by natural killer cells makes this response an important natural defence mechanism against malignancies. The non-specific, rapid response of natural killer cells differs from the response of T cells in that T cells first need to recognise a specific antigen, in order to provide a specific immune response.
B cells, as part of their role in the adaptive immune system and specialising in providing the humoral immune response, carry out different modes of action (Figure 3).
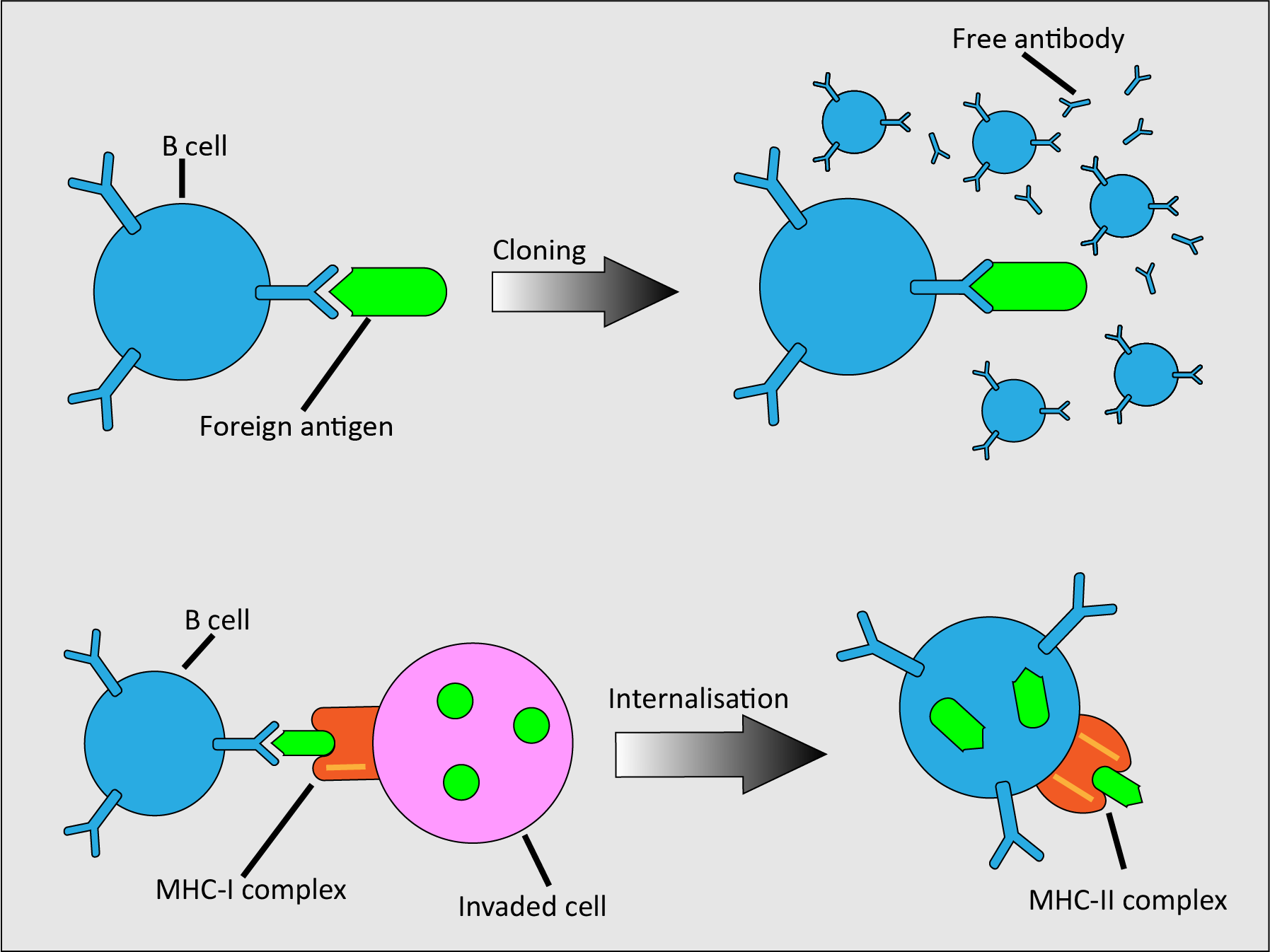
Individual B cells carry random membrane-bound antibodies on their surface. When one encounters a complementary foreign antigen, or when activated by T cells, the B cell clones itself. Most of these clones are effectors that release free antibodies to attract phagocytes (a type of cell that is able to engulf and digest harmful particles and cells; see below), but some are memory cells. Memory cells are long-lived B cells that maintain the ability to provide a specific immune response to a particular antigen long after the first encounter. B cells can also respond to infected cells; these cells present foreign antigens on their MHC-I protein. When B cells encounter them, they internalise the foreign antigens and present them in an MHC-II complex, ready for recognition and action by helper T cells (see below).
T cells, as part of their role in the adaptive immune system and specialising in providing the immune response dealing with infected cells, also carry out different modes of action (Figure 4).
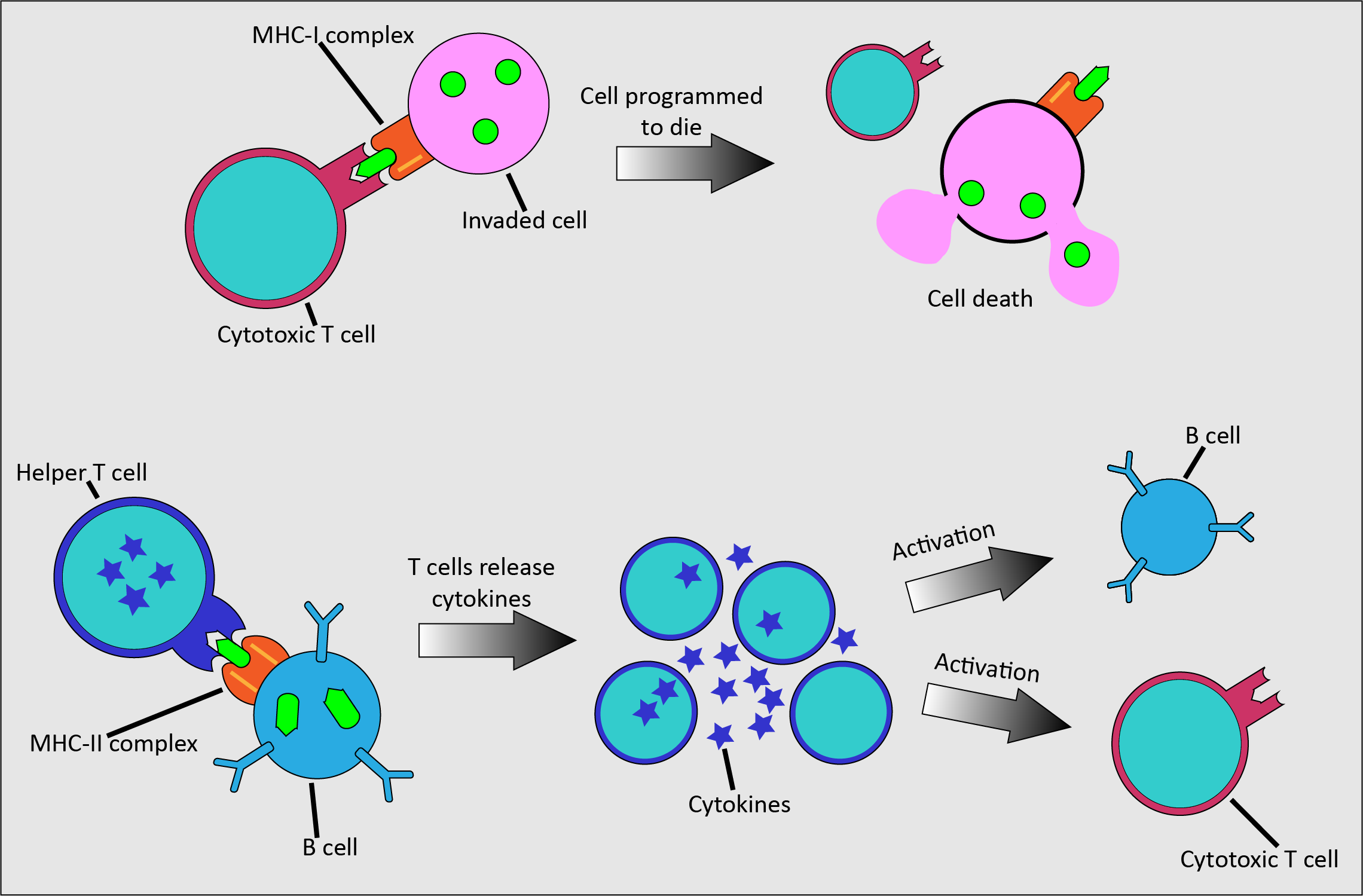
Cytotoxic T cells kill infected cells by releasing toxic chemicals once activated by binding to the MHC-I complex of an infected cell. Helper T cells are fundamental across all activities of the adaptive immune system. They bind to MHC-II complexes of infected cells and release cytokines. These chemical messenger proteins initiate the activation of antibody production in B cells, have a role in activating cytotoxic T cells, and help to attract macrophages to engulf and remove harmful particles.
Phagocytes are another part of the innate immune system. They are specialised cells that can be found everywhere in the body, able to engulf and digest harmful particles (phagocytosis) and to provide a rapid response. The most common type of phagocyte in humans are neutrophils, which circulate in the bloodstream and are early responders. Macrophages are another type of white blood cell found almost everywhere in the body; these cells are the ultimate scavengers and specialise in cleaning up dead cells and other cell debris.
Strategies to exploit the immune system for therapy of malignancies
A number of different strategies to exploit the immune system in combatting malignancies hold promise for future clinical use:
- monoclonal antibodies;
- checkpoint inhibitors;
- adoptive cell transfer;
- iNKT cells;
- vaccines.
Not all strategies will work across the range of malignant diseases. For example, head & neck malignancies typically show strong immunosuppressive properties, different from most other malignancies. Attempts at interfering with the complex immune system are also likely to be plagued by unintended, serious adverse effects. Another hurdle currently is the difficulty in predicting who will benefit from which treatment approaches, as there have not yet been reliable biomarkers identified for purposes of evaluation of treatment success and failure. This is a significant aspect for providing meaningful and helpful immunotherapies. Typical criteria to assess the ongoing treatment with conventional therapies, for example chemo- or radiotherapy will look at say, tumour shrinkage as a measure of success and effective treatment (whereas surgery will only regard complete excision with a pre-defined margin of normal tissue as ‘success’). That will typically not work easily in immunotherapies because the paths and timelines of reaction to treatment will be different from those in conventional treatment schemes.
Monoclonal antibodies work by mimicking natural antibodies, meaning that they support the immune system and that there can be many different types of monoclonal antibodies. However, they must have a specific target, such as a specific antigen, which they can bind to. Each monoclonal antibody will only effectively interact with one specific target (Figure 5).
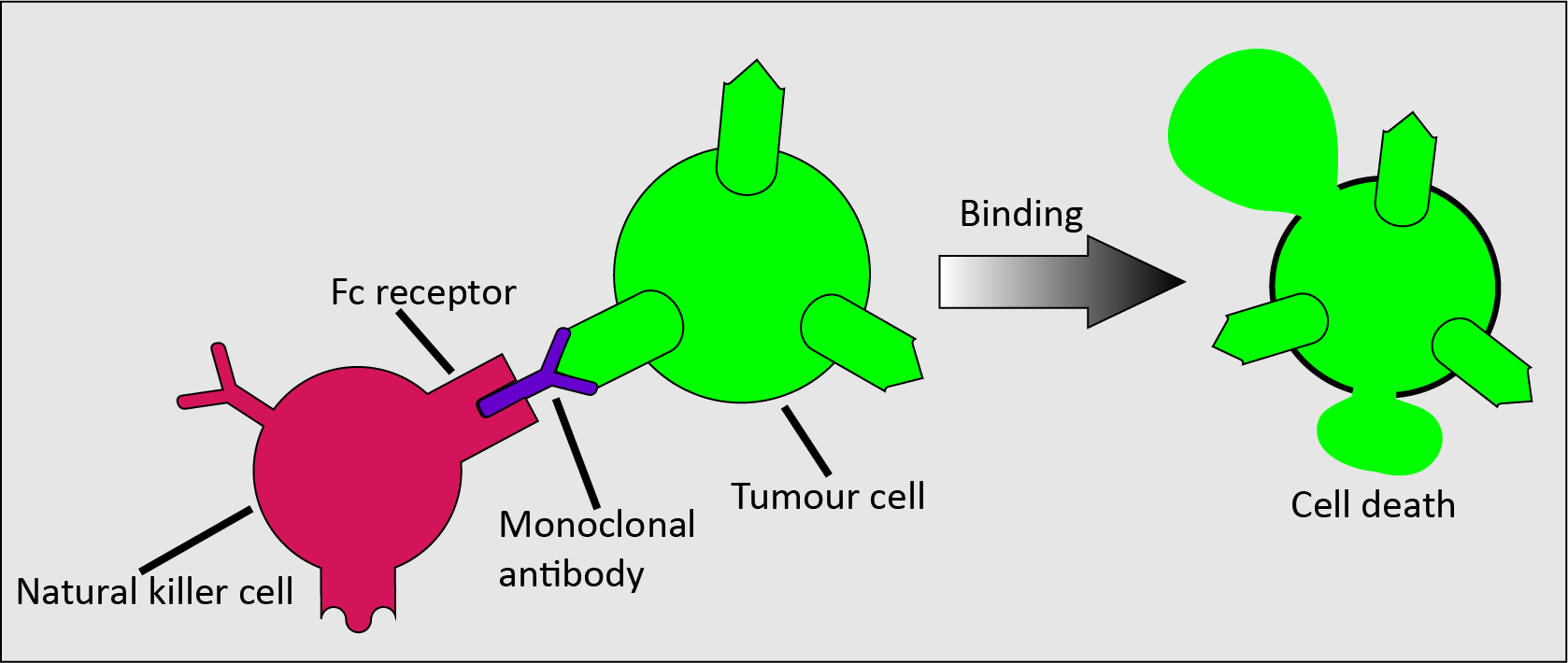
The main mode of action for cancer treatment by means of monoclonal antibodies involves the design of monoclonal antibodies that can bind to particular tumour antigens to form a complex. This complex is recognised by an immune effector cell (such as a natural killer cell) which binds its Fc receptor to the Fc domain of the antibody; this binding causes tumour cell death (the Fc receptor is common protein motif on the surface of many cells involves in the immune system; the Fc surface domain specifically binds to complexes such as the one made up of a monoclonal antibody and a malignant cell). This process is called antibody-dependent cell cytotoxicity.
Figure 6 schematically depicts another possible mechanism which does not involve the immune system, complement-dependent cytotoxicity.
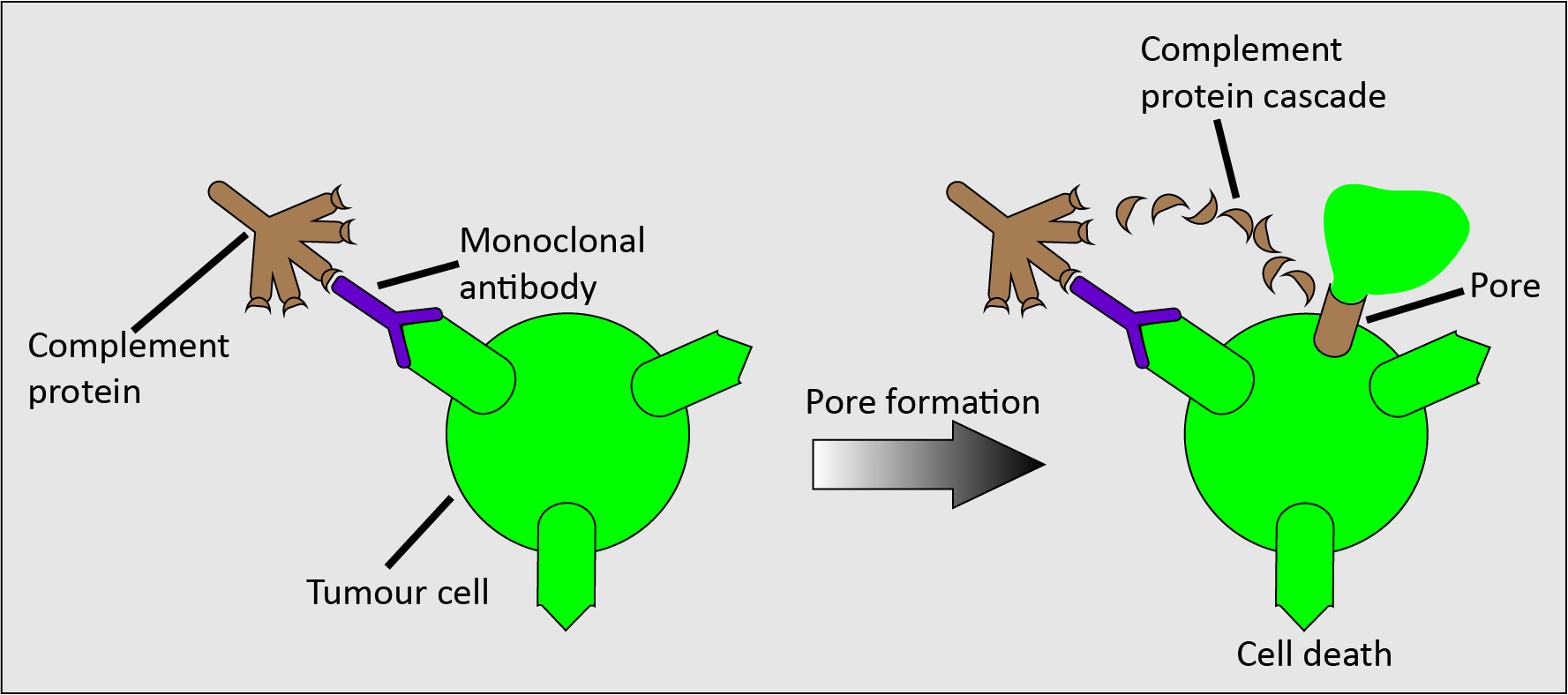
In complement-dependent cytotoxicity, a complement protein binds to the monoclonal antibody-tumour antigen complex, triggering a cascade of complement proteins that results in the formation of a pore on the cell surface (the membrane attack complex), so causing cell lysis (dissolution of the cell).
Monoclonal antibodies could also be attached to a non-toxic drug, which then guides them to the tumour antigen where the drug is subsequently converted (by the antibody) to the cytotoxic drug. This strategy may be useful to reduce the systemic toxicity of cytotoxic drugs in general.
A monoclonal antibody drug that has been approved for use in treating head and neck cancers is cetuximab. It targets the epidermal growth factor receptor, which when stimulated leads to cell growth. The epidermal growth factor is overexpressed in many malignancies. Cetuximab binds to the epidermal growth factor receptor with a higher affinity than the normal epidermal growth factor proteins, so blocks them from binding and therefore slows tumour cell production.
Other monoclonal antibody drugs that have shown promise but that are not yet used for treating head and neck malignancies (and may not be suitable anyway), are panitumumab, trastuzumab, and rituximab.
Checkpoint inhibitors can be considered as a form of monoclonal antibodies.
The immune system has mechanisms for turning off T cells, inhibitory checkpoints, that ensure that the immune system does not deliver an autoimmune response. Checkpoints normally switch on and off the T cell proteins as required for an adequate immune response. However, many malignancies produce large amounts of the ‘on’ protein for an inhibitory checkpoint, meaning that T cells are inhibited and will not attack the malignant cells. Checkpoint inhibitor drugs disrupt these signals sent by malignant cells and therefore expose them to an immune response (Figure 7).
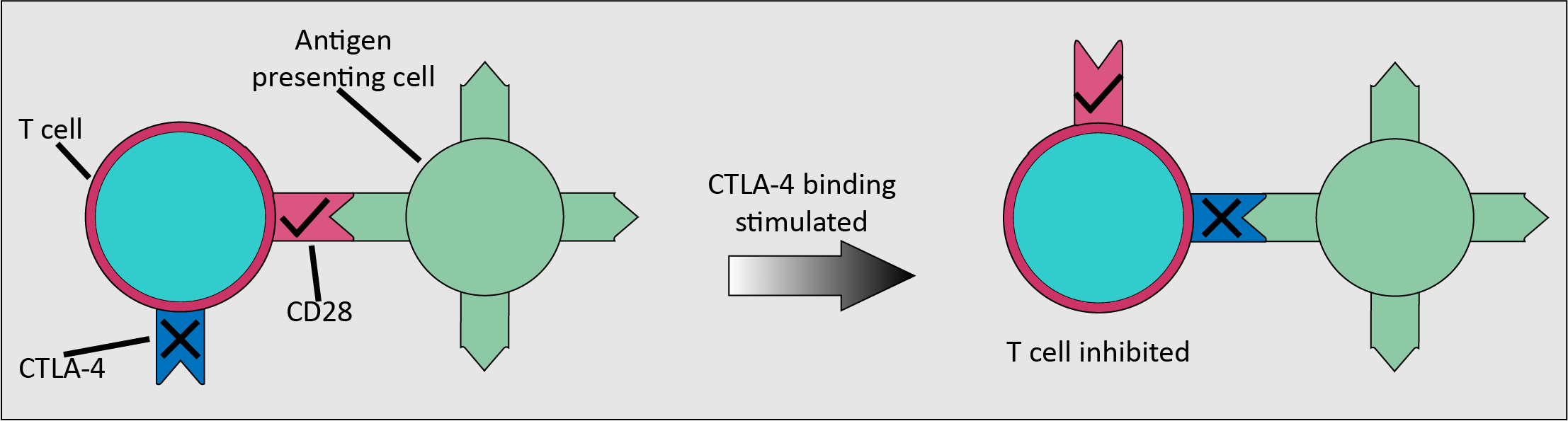
CTLA-4 is an inhibitory checkpoint receptor, found on newly activated and regulatory T cells, along with the stimulatory receptor CD28. The binding of CD28 to ligands on an antigen-presenting cell stimulates CTLA-4. CTLA-4 has greater affinity for binding to the ligands than CD28, so the T cells are inhibited. Ipilimumab is a drug that binds to CTLA-4 so blocks tumour-produced proteins from binding, therefore allowing an enhanced immune response. Ipilimumab has been used with some success in the treatment of metastatic melanoma.
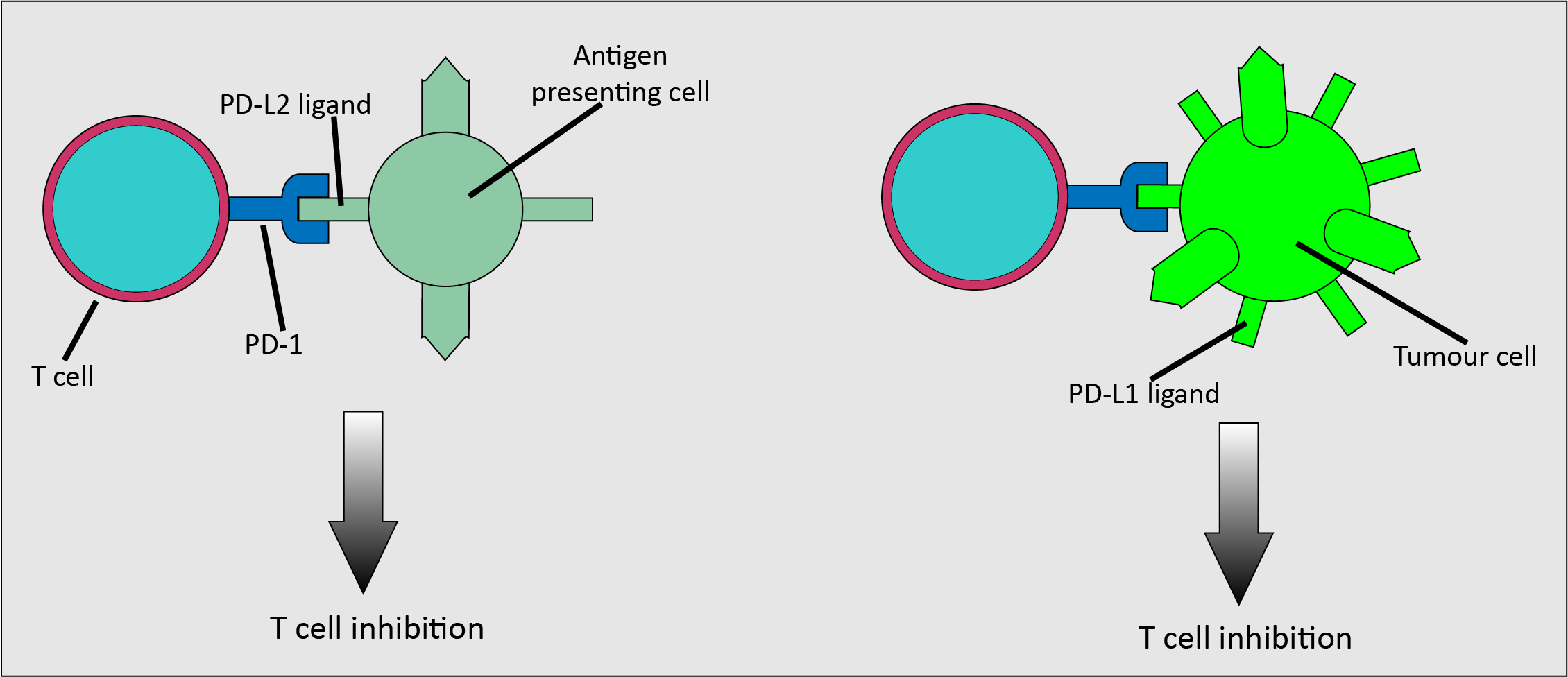
Another inhibitory receptor is PD-1, which is expressed on activated T cells. It is activated by the PD-L2 ligand found on antigen-presenting cells, and the PD-L1 ligand which is found on various cells types (Figure 8). Importantly, it is upregulated on many malignant cells, meaning that malignant cells can use the inhibitory interaction as a method to evade an immune response. Pembrolizumab and nivolumab are drugs that inhibit the PD-1/PD-L1 pathway. These drugs have been used to treat metastatic melanoma which meets specific marker criteria, but not as a primary treatment. Oral mucosal melanoma is treated surgically, although if it recurs or spreads, a systemic approach may be viable.
The underlying principle of adoptive cell transfer is to transfer tumour-infiltrating lymphocytes with anti-malignancy activity to a patient. The lymphocytes originate from the patient themselves. The lymphocytes are extracted from the tumour cells, the tumour-infiltrating lymphocytes that show the highest affinity for the malignant cells are isolated, this population is expanded and then re-injected.
One of the main forms of adoptive cell transfer is chimeric antigen receptor (CAR) T cell therapy, which involves gene modification. T cells are removed (apheresis) and isolated, then altered to CAR T cells by inserting the CAR gene, so that they can then recognise and target proteins on malignant cells more effectively than normal T cells. For this treatment to be most effective, the target antigen should be uniformly expressed on the tumour cells, but not on healthy cells. Adoptive cell transfer is a fairly new approach. For example, in the UK it was approved in 2018 for the treatment of some forms of childhood leukaemia and lymphoma in adults. For the time being, adoptive cell transfer treatment schemes have a number of serious adverse effects (cytokine release syndrome), including neurotoxicity.
Invariant natural killer T cells (iNKT cells) are a subset of innate-like T cells that recognise non-peptide antigens presented on the CD1-d protein. They are some of the first cells activated in an immune response and could be important in regulating the immune response to malignancies as well as to antigens. This is thought to be another promising avenue for further research. It has been demonstrated that the number of these cells is reduced in many cancer patients and this finding is correlated with overall survival rates. It is thought that lower numbers of iNKT cells indicate defects in the immune response.
Vaccines represent an area of much ongoing research. There are many different types of vaccine being looked into as they all have different targets, but the general aim is to stimulate or restore an immune response against malignancies. A promising area of research is their application as a therapeutic measure, aiming at delaying tumour growth and possibly achieve shrinkage. Sipuleucel-T is a therapeutic vaccine that was approved in the US for the treatment of prostate cancer in 2010; it is not approved in the UK and the evidence base for its effectiveness is slim. Some isolated spectacular initial responses have been seen in trials in head and neck cancer but none have demonstrated a long term benefit.
A more traditional view to, and application of, vaccines, to prevent some viral infections, is relevant in a maxillofacial surgery context. The human papilloma virus (HPV) is known to have some high-risk strands that are associated with causing oropharyngeal malignancies (amongst other malignancies).
Whilst vaccination against HPV infections (especially the high-risk strands 16 and 18; plus strands 6 and 11) is not a therapy to treat oropharyngeal cancers, this vaccination can prevent the development of these malignancies in the first place very effectively. The majority of HPV related malignancies afflict women (a leading cause of cervical cancer), so the initial vaccination schemes were only offered to girls. It is to be welcomed that in many countries these schemes have been modified and are now offered to boys and girls. Not only is this prevention by vaccination for all cheaper than the treatment costs in the absence of such vaccination schemes – HPV vaccination also prevents much suffering.