Bleeding
The basic components of blood
Blood is a mixture of several basic components, each component with specific physiological tasks, considerable overlap of regulation mechanisms and some degrees of ‘job sharing’.
The liquid component of blood is plasma. It makes up a little more than half of the volume of blood and mainly consists of water. Water-soluble compounds are dissolved in plasma. These chemical components include some vitamins, glucose, electrolytes (ions, including Na+, K+, Ca2+, Mg2+, PO43-, HCO3-), some proteins and hormones. Some of the water-soluble proteins in plasma help to carry non-water-soluble, large molecules (such as cholesterol) around the circulatory system. The electrolytes dissolved in the plasma act as the buffer system that maintains the body pH value near neutral.
The erythrocytes (red blood cells) are responsible for the oxygen (O2) transport by blood from the lungs to all body tissues, in the process known as aerobic respiration. The erythrocytes carry out this task by making use of a large (typically a tetrameric complex in adults) protein, haemoglobin (Figure 1 shows the crucial centre part, the ‘working unit’ haem of the molecular haemoglobin arrangement). Haemoglobin makes up the majority of the mass of the red blood cells and increases the blood’s ability to transport oxygen by nearly two orders of magnitude, compared with the amount that could be circulated by simply dissolving O2 in the plasma alone.
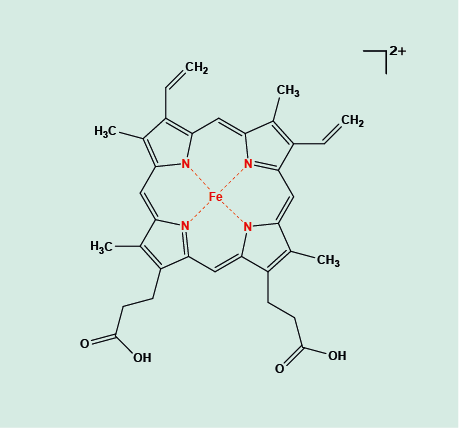
Figure 1 depicts the central Fe2+ unit held in its surrounding porphyrin ring. The Fe2+ ion is the bonding site for reversible attachment of O2 molecules. This attachment happens in the smallest blood vessels (arterioles) of the lungs. From there, the blood stream carries the oxygenated haemoglobin molecules in the red blood cells to all destinations in the body where energy-intensive processes happen. At such sites / cells, the O2 molecules are released from the red blood cells and are used in a chemical process to make the ‘energy storage molecule’ ATP (adenosine-triphosphate; see Figure 2). ATP is used by many energy-intensive processes in cells: releasing one of its three phosphate units to form ADP (adenosine-diphosphate) releases energy. The waste product of this aerobic metabolism powering the body is another gas, carbon dioxide, CO2. Haemoglobin also binds and removes this waste product from its production sites. This is not in competition with haemoglobin binding O2 molecules because CO2 attaches to the protein (globin) part of the haemoglobin complex. Other small molecules, notably carbon monoxide, CO, with a very high affinity to the haem Fe2+ site bind competitively with O2 and hence are toxic to the body (this is how someone can apparently be pink and dead from anoxia). The amount of O2 molecules bound to haem is proportional to the partial pressure of O2 in the atmosphere: the higher the partial pressure, the greater the amount of bonded O2 molecules. This physical principle forms the basis of hyperbaric oxygen therapy, HBO, aiming to improve oxygen delivery to body tissues.
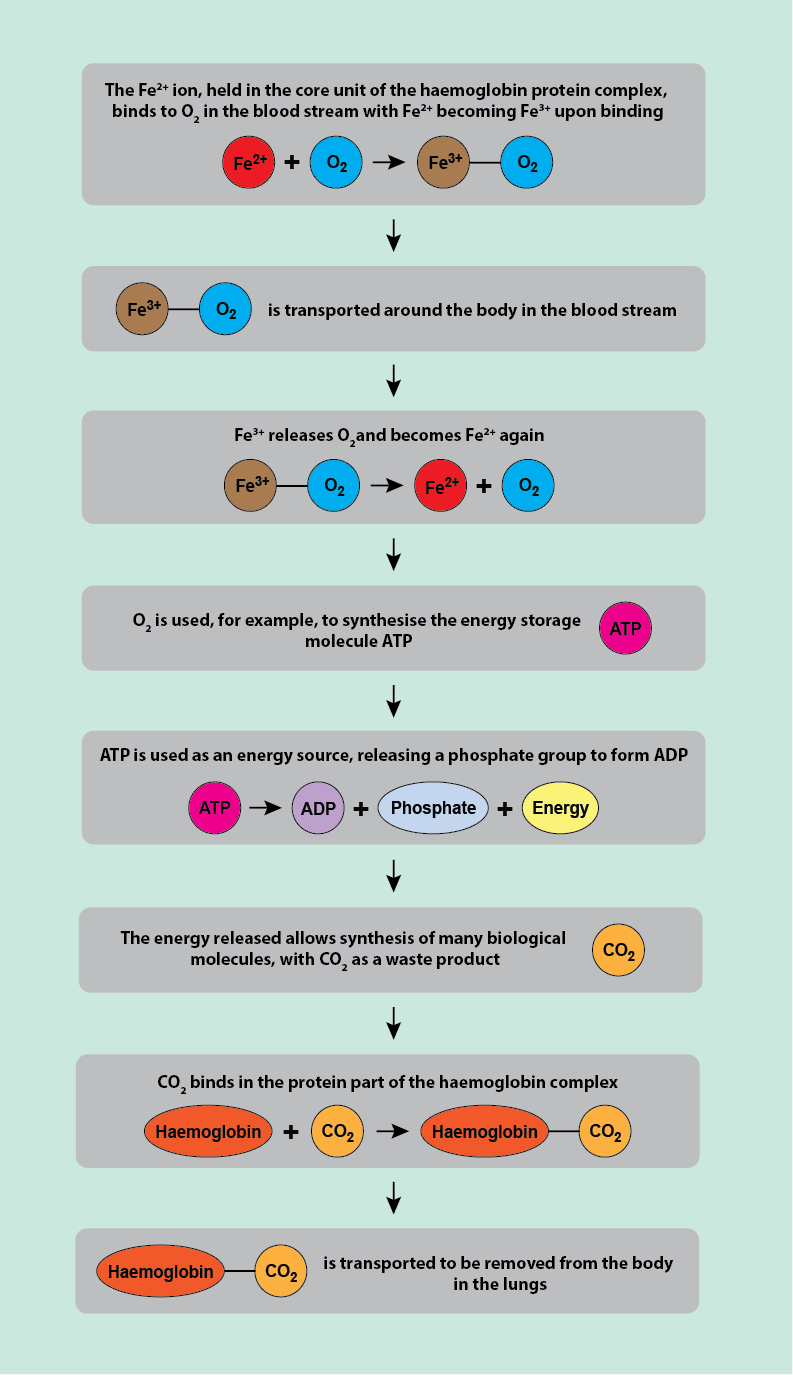
Red blood cells are small cells without a nucleus and circulate in large numbers in the blood system, with each red blood cell carrying of the order of 100 to 200 million haemoglobin molecules. These large numbers are necessary to satisfy the body’s need for oxygen / energy in all its tissues. Red blood cells typically have high turn-over rates and get replaced every three to four months. New red blood cells are constantly produced in the bone marrow where also the iron from old haemoglobin molecules is recycled. The haem units are broken down in the liver and are excreted in the bile.
The leukocytes (white blood cells) are larger cells with a nucleus but do not carry haemoglobin molecules. The different types of white blood cells originate from stem cells in the bone marrow and they constitute only a small fraction of the blood volume. Nevertheless, the different types of white blood cells are at the heart of the body’s immune system and its response to injury and infection. White blood cells can enter the space between cells (interstitial spaces) by escaping through capillaries from the intracellular space, important to counteract infections in a range of tissues.
The thrombocytes (platelets) are small cellular fragments originating from some specialised cells (megakaryocytes) in the bone marrow. Platelets circulate for only a few days before they are processed and removed in the liver and spleen; platelets require constant replenishment. The role of platelets is essential in blood clotting, both in the initial immediate response to bleeding as well as carrying and releasing biochemical compounds (so called factors) that are essential in the initiation and regulation of blood clotting (see below).
The basic steps of haemostasis (stopping bleeding)
Haemostasis, stopping bleeding after injury by sealing of the injured wall of a blood vessel may be seen as a three-step process. The first step (primary haemostasis) is a rapid emergency response to stop or reduce further blood loss. This is followed by secondary haemostasis, the stage of formation of a blood clot that is stable enough to permit healing of the wound and the injured blood vessel. The final stage of the process, after healing, is called fibrinolysis and is the process that removes the then no longer necessary blood clot from the healed site of injury. This chronology of events is illustrated in the sketch in Figure 3.
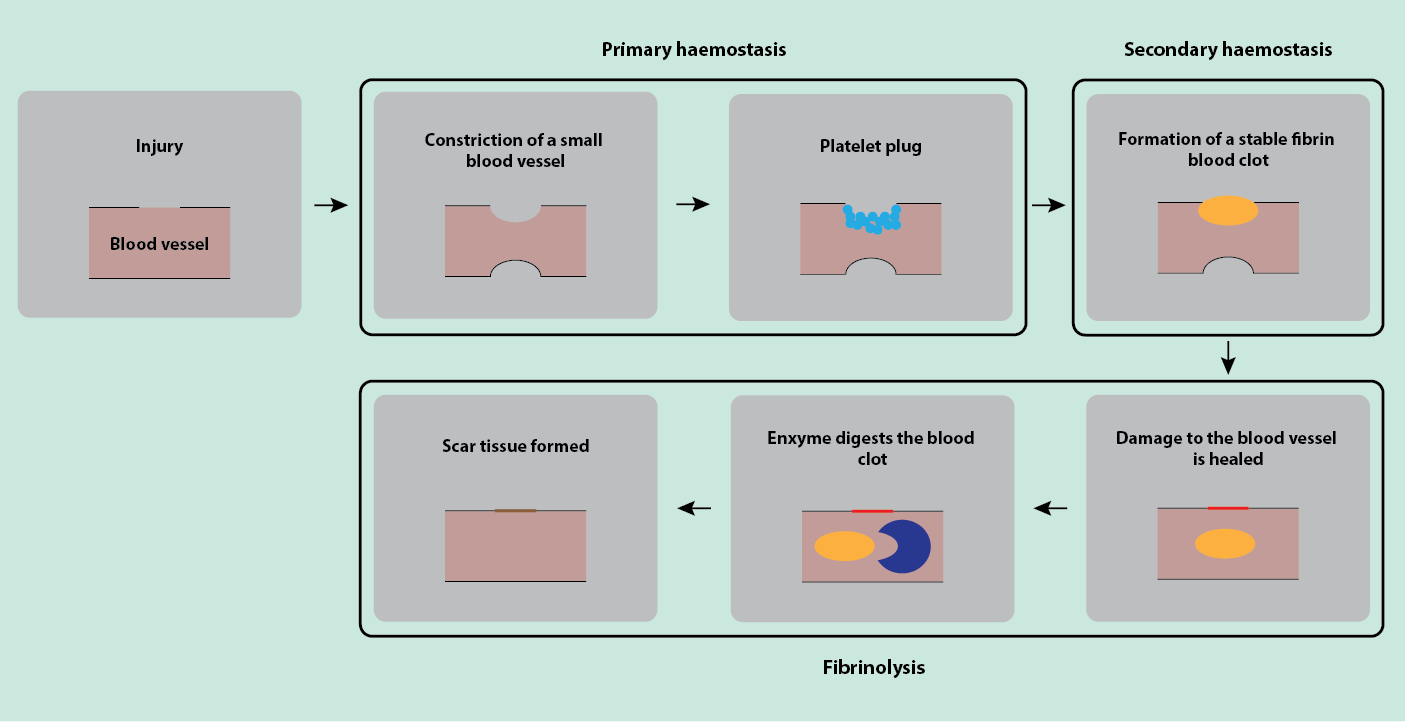
The haemostatic process is essentially maintained by three main players: the platelets, the lining (endothelial cells) of the blood vessels, and a range of proteins with blood-clotting properties. In the absence of injury, the role of endothelial cells is actually to prevent the clotting of blood and to ensure a smooth blood flow; proteins with blood-clotting properties circulate in the blood plasma in non-active form but are always ready to be activated in case of injury.
Primary haemostasis follows injury immediately. It involves constriction of the small blood vessel to reduce blood flow to the site of injury and the rapid formation of a platelet plug to stop haemorrhage. This initial plug formation is a process that materials scientists call a phase transition, here a transition from the liquid phase to a gel phase.
The formation of this initial platelet plug follows the exposure of collagen fibres by the injury to the lining (endothelial cells) of a blood vessel. In an intact blood vessel the endothelial cells prevent blood-clotting and aggregation of platelets by the release of a range of chemicals such as heparin-like compounds or prostacyclin. After injury, such inhibitor compounds for aggregation and/or clotting are no longer released. The platelets stick to the collagen-fibre surfaces, then to each other, and release chemicals that lead to constriction of blood vessels. At this stage the platelets initiate the next stage in the process by releasing further chemicals that trigger the formation of a more stable blood clot by starting the formation of fibrin.
Secondary haemostasis stabilises and maintains haemostasis by supporting the formation of a stable fibrin / blood clot (coagulation). This is a much slower process than the initial emergency response and formation of a platelet plug. It involves a series of chemical reactions, each initiated by some messenger molecules (coagulation factors, of which approximately ten are currently known to play a major role in this process). The coagulation process involves another phase transition, the transformation of a soluble protein, fibrinogen, to an insoluble form, fibrin. Fibrin forms the stable backbone of the clot. This fibrin clot seals and protects the injury site of the blood vessel during healing. Fibrin formation is the result of a chain of commands to/from inactive plasma proteins. The first major step activates prothrombin -> thrombin (an enzyme); thrombin in turn triggers the activation of soluble fibrinogen -> insoluble fibrin.
Fibrinolysis is the final stage of this repair process. Once the damage to a blood vessel is healed, the clot is no longer necessary and is dissolved. The fibrin clot is digested by another enzyme, plasmin, into small fragments. The inactive form of plasmin, plasminogen, is synthesised in the liver and gets incorporated into the fibrin network of a clot. There it is converted to its active form, plasmin. Eventually, the fibrin clot is replaced by regular, permanent scar tissue which consists mainly of collagen.
Another basic mechanism to stop haemorrhage is equalising of the pressure inside and outside of an injured blood vessel. Bleeding continues as long as the damaged site remains open and the pressure inside the blood vessel is higher than that outside. The initial reaction to reduce the blood flow to the injured site by constriction of the (small) supply vessels reduces the intravascular pressure. The external pressure may be increased by blood that is trapped in the vicinity of the site of injury (haematoma). It may, of course, be simulated by direct pressure on a wound – the mainstay of first aid.
The relative importance of the various mechanisms to stop bleeding depends on the site and the scale of a vascular injury. Bleeding from small vessels is usually stopped by the formation of a platelet plug. Stopping bleeding from a larger vessel usually requires the formation of a blood/fibrin clot. Blood loss following injury to the largest vessels, in particular arteries, is usually not stopped by the aggregation of platelets and the formation of a blood clot. Here a massive drop in pressure (shock) is the mechanism to arrest blood loss. Unfortunately, that equates to failure of adequate O2 delivery at a cellular level – the metabolic consequence of hypovolaemic shock. This precipitates systemic dysfunction unless rapidly reversed.
The opportunities and causes for disturbances and malfunctions of the blood clotting process at every stage are numerous. There can be over- as well under-activities. Most of the resulting conditions, signs and symptoms can be successfully managed by a range of treatment options. Since all these aspects of bleeding are relevant in all areas of surgery, oral & maxillofacial surgery included, we next discuss on the following page malfunctions and pathologies of the various blood clotting processes, possible remedies and management (including surgical interventions), and interference with surgery and/or recovery from surgery.