Clinical application (Radiotherapy)
Teletherapy (external beam radiotherapy)
This is the most widely used method. The machines used are linear accelerators (LINACS) that use microwave technologies to accelerate electrons such that after their collision with a heavy metal target a high-energy X-ray (1-25MeV) photon beam is produced. This photon beam is precise in definition, direction and depth allowing careful planning in terms of delivery. Delivery planning is almost always aided by CT scans. This is schematically illustrated in Figure 1.
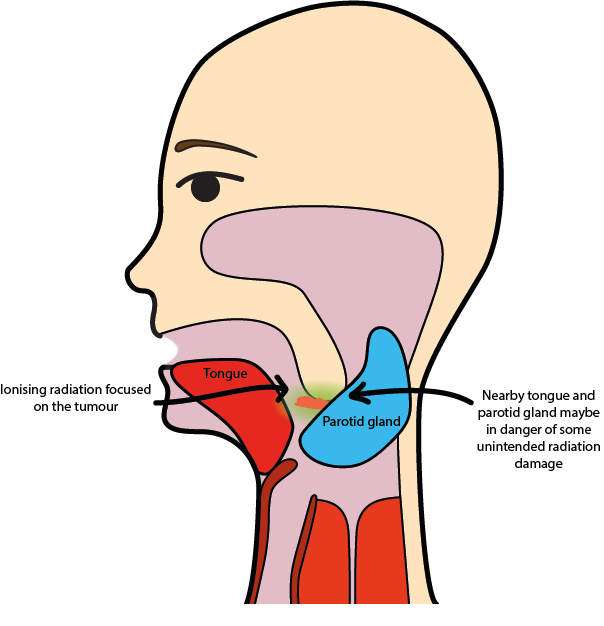
The precise location of the tumour and nearby healthy structures that need to be protected from radiation as far as possible are determined before delivery starts.
For each session the patient is immobilized in a clear custom made plastic shell which must fit accurately over the face in order to ensure consistent and reproducible delivery in each session (see Figure 2). The need for the mask to fit can create significant delays if radiotherapy is postoperative and full resolution of postoperative swelling has not occurred, or even worse there is flap failure delaying the optimum window of treatment (ideally starting around 6 weeks postoperatively) or creating further anatomical changes.
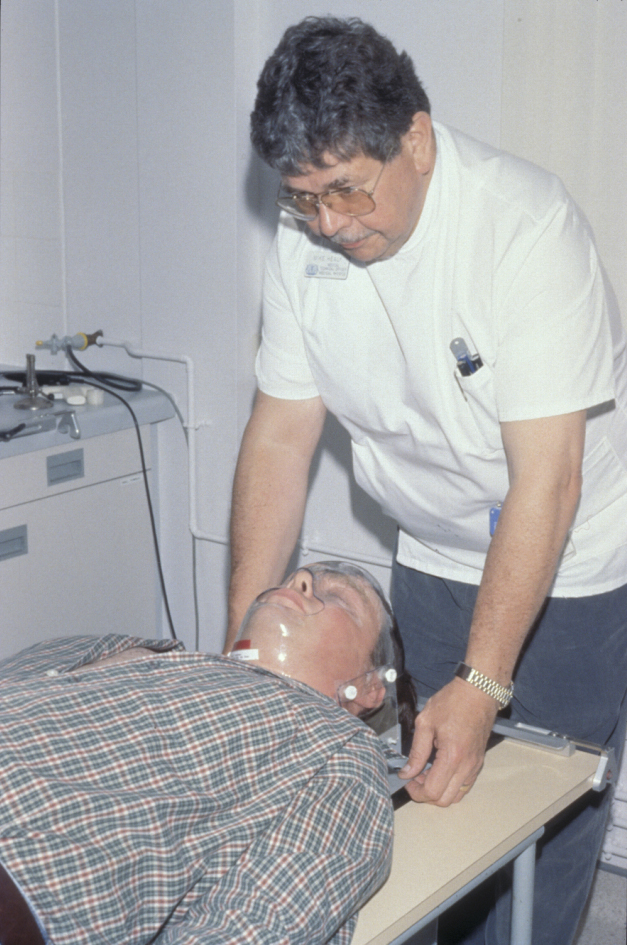
Multiple beams are used to maximize delivery to the tumour target volume yet minimizing the effect on adjacent healthy tissue such as salivary glands, spinal cord and lens of the eye. The accuracy of this process is improved by CT planning and sophisticated irradiation schemes such as intensity modulation (IMRT, see below).
There are several regimens with variable dosage and fractions (a fraction is a single dose delivered) used. The optimum treatment time and number of doses remains controversial and dependent on the volume irradiated. A common regimen for head and neck cancer is around a total dose of 65 Grays (a Gray is energy absorption of 1 Joule/kg) delivered as multiple fractions over 35 days. The optimal treatment of radiotherapy is balanced between achieving control of disease and too much irradiation causing necrosis or significant late effects.
The therapeutic effect of radiotherapy can be reduced by delays between fractions (allows tumour cells to repair and repopulate), the patient continuing to smoke and where radiotherapy is delivered after surgery (adjuvant radiotherapy, see below), relative hypoxia (insufficient oxygen levels in tissue) and a long delay between surgery and start of radiotherapy.
Brachytherapy (interstitial radiotherapy)
The principle of this method is to deliver ionizing radiation by inserting radioactive source(s), usually removable iridium-192 implant wires, into the tumour using slotted guide needles or plastic tubing. This method will deliver a high intensity of radiation into its immediate vicinity with a rapid fall in dosage in the surrounding tissue (radioactive isotopes such as iridium-192 radiate monochromatic high-energy photons (gamma rays) due to their radioactive decay). The number of needles inserted will be calculated according to irradiation volume and dosage required. The needles are inserted manually or using a remote computerized loading system (to avoid staff irradiation) under general anaesthesia with radiological checks. As the patient is radioactive, he or she will be kept in a protected and isolated environment for the duration of the treatment. A common regimen will involve delivery of a total dose of around 65-70 Grays over 6-7 days.
This technique is not suitable for patients with poor compliance or claustrophobia. Tumours very close to or involving bone are also unsuitable for treatment using this approach. It is mainly used for small tumours with the key advantage of good functional outcome. It is an effective treatment modality but does not address the neck. The post treatment area is often necrotic and very painful. This creates real difficulty in the first few months of distinguishing between persisting disease and radionecrosis.
Adjuvant and neoadjuvant therapy
The rational for postoperative (adjuvant) radiotherapy is that a small volume (microscopic) of malignant cells may be left within the surgical bed through spillage or incomplete excision in the margins. Where frank tumour is present, it is less effective and in such instances, further resection is advocated. However, this may not always be possible (due to proximity of vital structures, patient unable or unwilling to have further surgery or flap reconstruction in situ). It has been shown that irradiating the operated tumour bed or neck will help reduce the chances of recurrence by some 50%. Ideally this is carried out within six weeks of surgery.
Neoadjuvant radiotherapy or chemoradiotherapy is treatment given to reduce the bulk of a tumour before the definitive treatment. In its original sense it was given to shrink a tumour to make it possible to resect surgically. As the margins of normal tissue required for surgical excision with curative intent remain the same, the principal role in head and neck cancer is to assess the response of the tumour. An example would be giving chemotherapy initially and following this with radical chemoradiotherapy if there was a good initial response to the chemotherapy, or even initial palliative doses of radiotherapy but increasing to a radical dose with curative intent if the response was promising. In these cases surgery is regarded as “salvage” in the event of recurrence of, or persisting, disease.
Side effects of radiotherapy
Unlike surgery where a lot of the side-effects are manifested quickly, radiotherapy’s side effects build up gradually. These can be divided into general, acute and delayed effects (see Table 1 for a summary).
Acute side effects | Features | Comments |
---|---|---|
General | Tiredness, lethargy, fatigue | Systemic effects of treatment |
Mucositis (sore mouth) | Erythema, exudates formation, ulceration |
One of earliest side effects to show due to rapid (12 days) mucosa epithelial turnover. Takes several weeks to heal after completion of radiation. |
Skin reactions | Erythema, desquamation | Slightly later (21 days). Healing similar to mucosa. |
Hair loss | In line of radiation beam | Usually temporary but can be permanent |
Loss of taste | Some change noticed |
Temporary as taste buds are fairly radio-resistant. Mechanism due to salivary changes? |
Xerostomia (dry mouth |
Reduced saliva production Sticky saliva Dental caries |
Salivary glands are sensitive to radiation. A common complaint despite attempts to reduce this side effect (shielding, avoiding beams, etc) Saliva substitutes, vigilant dental care including topical fluoride important |
Infection | Acute bacterial and fungal (candida) infections (thrush) |
Antifungal agents Chlorhexidine mouthwash painful to use, best to avoid. |
Late side effects | Features | Comments |
Ischaemia & fibrosis | White atrophied scarred tissue with telangiectasia, dry skin and mucosa |
Hypocellularity, hypovascularity Hypoxaemia |
Chronic ulcers, slow or poor healing |
Hypocellularity, hypovascularity Hypoxaemia |
|
Radiation caries |
Classically cervical caries, widespread |
Due to brittle enamel, poor saliva protection, sticky sugary diet, poor hygiene secondary to treatment |
Exposed bone, can be painful and progress to pathological fractures, dehiscence, halitosis. |
Prevention is better than cure – eliminate dental sepsis sources before irradiation, avoid trauma (e.g. ill-fitting dentures), atraumatic extractions in high risk teeth and possibly use hyperbaric oxygen (HBO). Treatment can be difficult. Sequestrectomy, HBO and radical surgery used. |
|
Malignancy |
New malignancy developing in irradiated area years later |
Small but real risk |
Intensity Modulated Radiotherapy (IMRT)
Radiation delivery has evolved from conventional external beam irradiation with a two-dimensional delivery model to three-dimensional conformal radiotherapy aiming for a more selective radiation scheme. In a further advance, Intensity Modulated RadioTherapy (IMRT) has been a significant technological advance since it allows sparing of healthy tissue while delivering radical radiation doses to the target volumes. IMRT works by subdividing the delivered radiation into multiple beams by using multiple collimators. In this way the radiation intensity can be finely modulated over the entire irradiated area, with differently intense radiation delivered to different areas.
The potential of IMRT for sparing organs at risk has been demonstrated in patients with mixed (oropharyngeal and nasopharyngeal) head and neck cancers. The multi-centre study PARSPORT that compared parotid sparing IMRT with standard radiotherapy in patients with oropharyngeal and hypopharyngeal cancer showed a significant reduction in xerostomia in the IMRT group at one year post-radiotherapy. IMRT has not yet been shown to significantly improve tumour control or patient survival compared with standard radiotherapy (two- or three-dimensional irradiation modes). The only randomised controlled trial comparing IMRT versus 3DRT reported to date, PARSPORT, had too small a sample size and too short a follow-up period to investigate any differences in clinical outcome. IMRT also has disadvantages resulting from the complexity of IMRT planning and delivery process, and the possibility of errors in planning or delivery.
Other high-energy particle beams
Even if by far the most widely used, photons are not the only option that can be used for high-energy irradiation. Other principally suitable particles include electrons, neutrons and protons.
Other radiotherapy beams used include electrons for irradiation of superficial tumours. The penetration depth of electrons (6-20MeV) in tissue (like in any other matter) is limited to a few cm. Beyond this depth the energy of the electrons very rapidly drops off due to multiple scattering events, thus causing less collateral damage but rendering it ineffective for the irradiation of tumours further away from the body surface). Linear accelerators used for photon-based radiotherapy are often suitable, with very minor modification of the set up, to deliver an electron beam instead of a photon beam. It is challenging to model and predict exactly the required electron irradiation scheme because of the heterogeneous nature and irregular shape of a human body.
Beams of accelerated neutrons went through a vogue but were seen to create much more morbidity for no greater benefit than photons. This may be related to the fact that high-energy neutrons cause direct damage to DNA in cells (different from the more indirect damage mechanism with photon irradiation). In principle this causes more severe and irreparable damage to tumour cells but at the same time also cause more severe (DNA) damage in healthy tissue.
Proton beam therapy is another modality. It is relatively new and setting up the equipment is very expensive. The proton beam has less scatter than conventional photon-based radiotherapy, due to the larger size of the particle. It is felt to be particularly effective for difficult to access tumours (skull base and paediatric cancers): protons can be accelerated to particular velocities, representing particular energies. This determines exactly how far they will travel in the body and where they will deposit their energy, tissue beyond this target area is only minimally affected. The use of proton beam therapy is restricted to highly specified cases in the UK. However, the technique is widely, some would say inappropriately, used in the US and parts of Europe. Maslow’s analogy of the hammer and the nail should always be borne in mind.
Chemoradiotherapy
Chemoradiotherapy involves cycles of up to 3 bouts of chemotherapy (often quite old fashioned platinum-based drugs in conjunction with conventionally fractionated high intensity (radical) radiotherapy.
There is real controversy about the suitability of these regimens as the unwanted effects include mortality at a greater rate than surgery (up to 3%), greater incidence of radiation mucositis, severe fibrosis leading to crippling trismus, dysphagia and radionecrosis. The improvement in survival claimed (8-15%) may simply have been a manifestation of the presence of the then unknown HPV driven head and neck cancers which are now recognised as being far easier to cure. The data is being revisited.