Mass spectrometry
We look into the basic working principles of an analytical technique widely used in chemistry, biochemistry and beyond - mass spectrometry. This analytical technique has recently been demonstrated to permit real-time distinction of healthy and malignant tissue in the operating theatre in breast and ovarian tumour surgery, when combined with diathermy (electrical cutting devices). The intention is to have a quick and reliable way to characterise the surgical margins as free of malignant tissue after tumour resection. The mass spectrometry-based technique is currently (2019) being explored and evaluated for similar applications in maxillofacial surgery.
Mass spectrometry is an analytical technique that allows the identification of unknown molecules, or confirmation that certain molecules are present in a sample, as well as quantification of different components in a mixture of chemicals. In many instances the composition of a sample can be analysed quantitively. In comparison with other analytical methods, mass spectrometry only requires small amounts of sample. Mass spectrometry is basically a brute-force method, and all mass spectrometers work by the same principles. Figure 1 depicts the schematic set-up of a mass spectrometer.
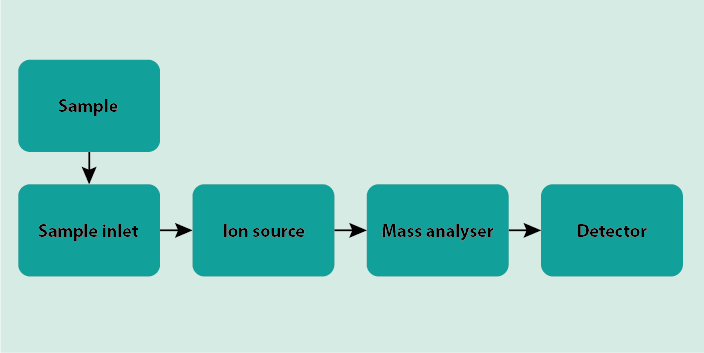
The sample is introduced into the mass spectrometer via the sample inlet. The sample then is ionised. ‘Ionised’ is an elegant wording for saying the molecules get smashed (in some way, by hitting the sample with electrons; there are ‘softer’ and ‘harder’ ways to bombard the molecules in the sample and the technique chosen depends on the application). At least some of the electrons which hold the molecule together are knocked out, and many of the evaporated molecules will fall apart into a number of ionic fragments. These fragments are characteristic of each individual chemical compound. This happens in the section of the mass spectrometer called the ionisation chamber, which is a vacuum chamber. Once ionised, the molecular ion and/or molecular fragments can be transported by electric or magnetic fields to the part of the spectrometer called the mass analyser (sometimes also called the ion separator). There are many different technical ways in which the mixture of different charged fragments at this stage can be sorted, according to their respective mass and/or charge. The choice of methodology depends on the particular application. Once sorted, the particles reach the detector where they are registered by recording the current or the charge induced by the various ions hitting the detector. Also for this detector part of a mass spectrometer a number of different technical solutions exist. These solutions usually require some form of signal amplification as there is only a very small number of molecular ions and fragments hitting the detector. The final output from the mass spectrometer is a chart that depicts all the components in the sample as characterised by their mass-to-charge (m/z) ratios.
Figure 2 depicts a schematic view of such a hypothetical chart for a particular molecule. From the ionic fragments and the molecular ion present, one can reconstruct the original molecule, if structure determination is the aim of the experiment.
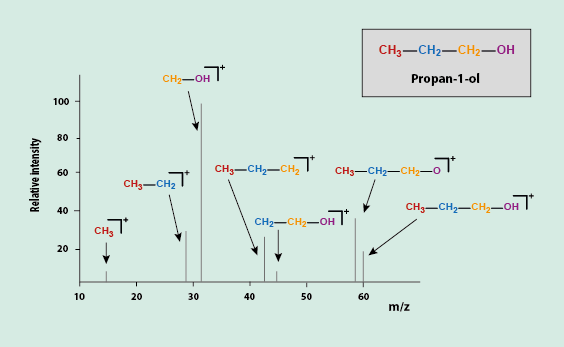
More commonly, analytical laboratory methods will be needed to analyse the composition of a mixture of chemicals (for example, in a blood or urine sample, or to distinguish genuine Bordeaux wine from fake versions, and many other applications), and to quantify the relative amount of the different components in the mixture (Figure 3).
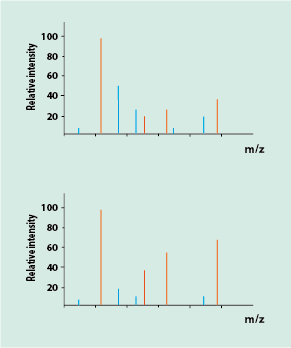
For this quantification based on relative peak intensities in the charts to work, the fragment patterns of the pure components in the mixture need to be known, so that the various peaks can be assigned to the different components. This is essentially the approach taken for surgical applications of mass spectrometry.
A particular version of a mass spectrometry (rapid evaporation ionisation mass spectrometry, REIMS) is used for surgical applications, in conjunction with an electrical surgical cutting device (diathermy) in the operating theatre. Figure 4 illustrates the general set-up.
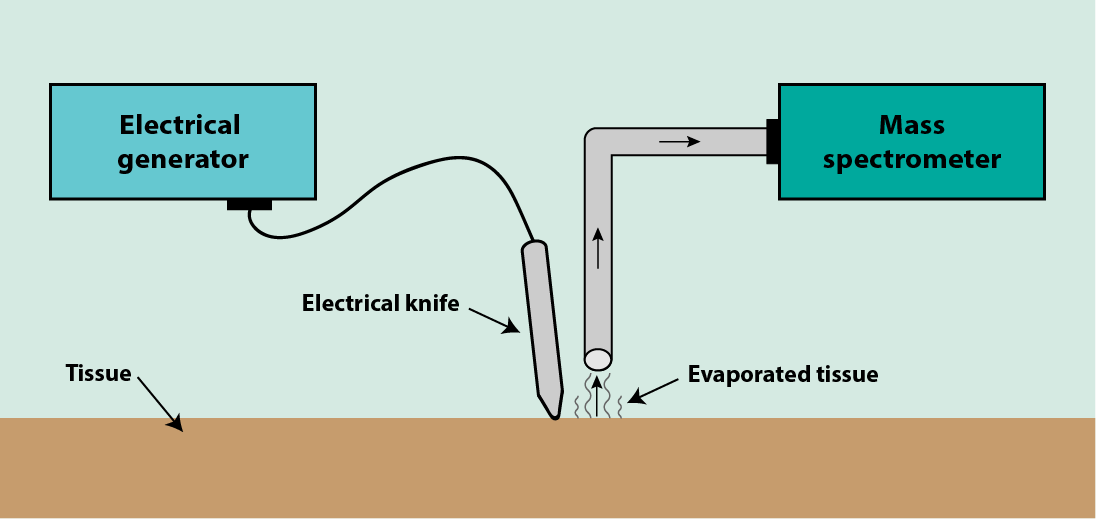
Cutting tissue with an electrical device strongly heats the tissue, producing ‘surgical smoke’ by evaporating tissue. The vapour is captured at the surgical incision site and is delivered to a (slightly remotely placed) mass spectrometer. The vapour consists of a complicated mixture of biological molecules that make up the cells of the evaporated tissue.
The general principle illustrated in Figure 3 is used to distinguish healthy and malignant tissues from their respective vapour composition. Both healthy and malignant tissues are composed of the same basic components. However, the different tissues differ in the relative abundance of these components. It has been demonstrated further that different types of malignant tumours can be distinguished by their mass spectrometry tissue fingerprint. One type of biological molecules that gives rise to such distinctive mass spectrometry charts of breast tissue are phospholipids. Phospholipid profiles in mass spectrometry charts were used with some success in breast surgery. Comparing the real-time data recorded in the operating theatre to data from a suitable data base, together with careful statistical analysis allows for rapid distinction between healthy and malignant tissues.
The approach has the potential to optimise surgical margins by ensuring that enough, but not more than necessary, tissue has been resected. Obviously, the method relies on diathermy being the principal cutting device; cutting with a scalpel does not produce surgical smoke. Diathermy may not in all circumstances be the optimal cutting device. Alternative real-time assessment techniques (such as Raman spectroscopy) that work with other cutting devices are also being explored. Further, the method relies on the identification of suitable molecular profile candidates to distinguish healthy and diseased tissues. In addition, a high-quality data base of profiles for the relevant types of tissues needs to be established in order to have a reliable statistical result.
These requirements need to be explored and examined for maxillofacial surgery, before diathermy in conjunction with REIMS can be established as a standard assessment tool for surgical margins in maxillofacial surgery. Mass spectrometry based methods will have to compare favourably with the use of frozen sections (comparator for immediacy of results), definitive pathology of the resected specimen (comparator for accuracy of results) and survival aspects, including quality of life (comparator for relevance). Alternative real-time assessment techniques (such as Raman spectroscopy) that work with other cutting devices are also currently being explored.
The scenario for oral and maxillofacial surgery may turn out significantly different from the situation encountered in the surgery of solid malignant tumours in other parts of the body, such as the breast.