Extreme temperatures
Contents
We outline the basic working principles of three different high and low temperature technical devices used in oral and maxillofacial surgery:
- lasers (light amplification by stimulated emission of radiation);
- diathermy and coblation;
- cryotherapy.
The discussion of the most common applications of these devices in oral and maxillofacial surgery includes respective advantages and disadvantages of the various techniques.
Laser (light amplification by stimulated emission of radiation)
The basic principles of a laser
A laser is an artificial source of light, not necessarily in the visible range of electromagnetic radiation. A laser differs from a normal light source in that it emits monochromatic (only one wavelength) and coherent (all waves are in phase) light waves (Figure 1). This type of coherent radiation can be exploited to generate intense and highly focussed beams of light with a precisely defined energy, a property that is highly desirable for a wide range of technical applications, including surgery.
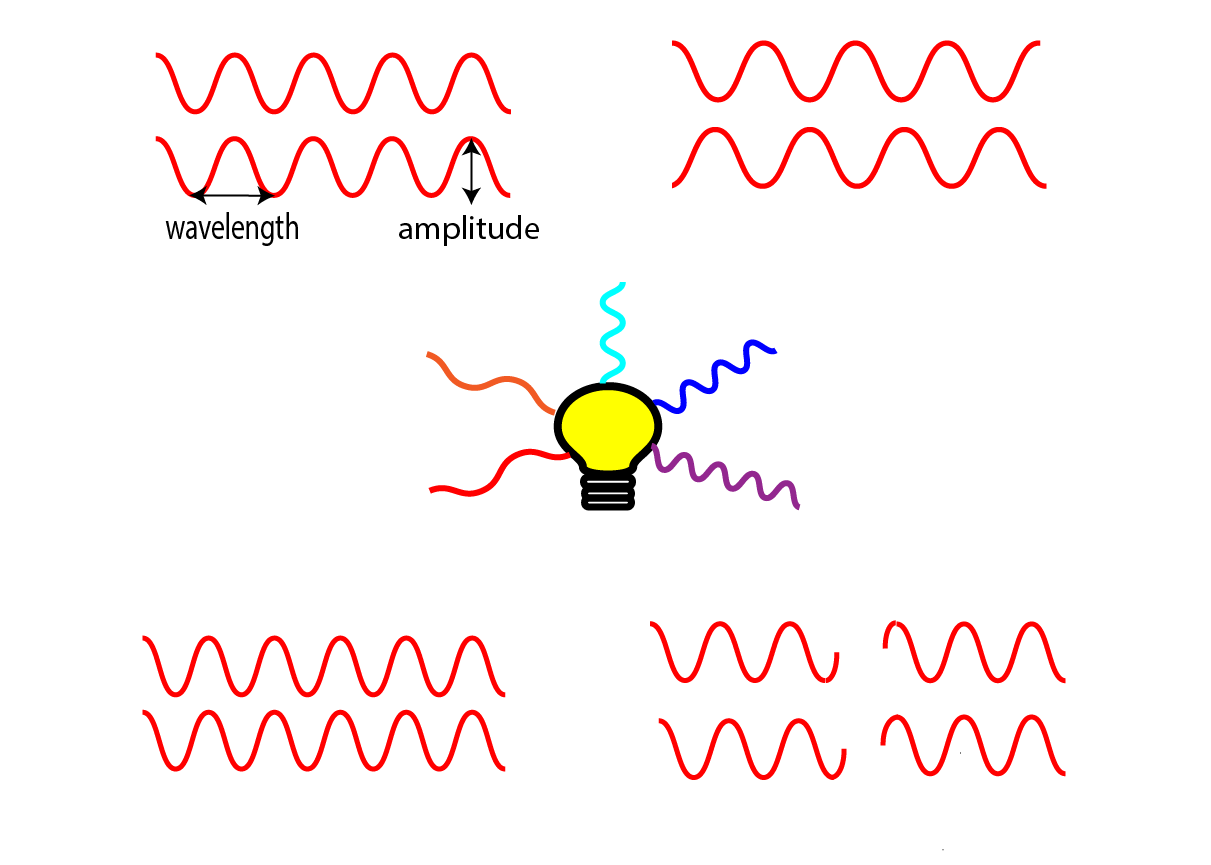
Most lasers can be operated in two different modes, continuous or pulsed. In continuous mode, an uninterrupted wave with a constant output is emitted. In surgical applications, this continuous-output, low-power (3 to 30 W) mode is very useful for cutting and ablation of tissues. In pulsed mode, an interrupted emission with set time periods for pause and emission allows for large amounts of energy to be delivered in a very short period of time (in surgical applications typically in excess of 100 W). This mode of delivery leads to mechanical disruption of tissues rather than thermal effects (laser lithotripsy, the breaking up of kidney stones or other calculi, being an example).
The laser phenomenon relies on a fundamental property of electrons, the particles that hold molecules and materials together by forming chemical bonds and by providing the negatively charged shell surrounding the positively charged nucleus of atoms. Electrons, like all other microscopic particles, obey the laws of quantum mechanics which require that electrons can only occupy certain discrete energy states / energy levels. Using the analogy of a staircase, an electron can be moved up from one step to the next by providing the appropriate amount of energy (absorption) to bridge the gap (Figure 2).
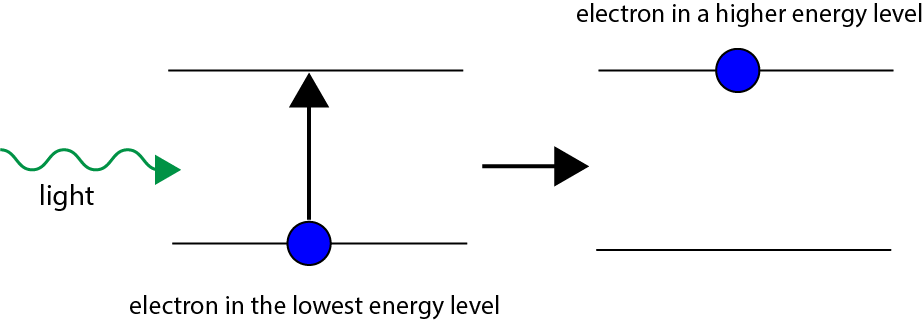
The reverse process of an electron transferring from a higher energy level to a lower energy level releases the corresponding amount of energy in the form of emitting light (photon). The return of an electron from a higher energy level to its ground state, lower energy level usually occurs spontaneously (spontaneous emission) without external influences or triggers. However, there is another mechanism of return to the energetic ground state, stimulated emission. This mechanism also leads to emission of a photon of energy corresponding to the energy gap, but the process is triggered / initiated by the interaction of the electron in its higher energy state with a suitable package of energy (typically in the form of light). This mechanism of stimulated emission of radiation (Figure 3) is the underlying principle that enables the construction of lasers with a very wide range of properties.
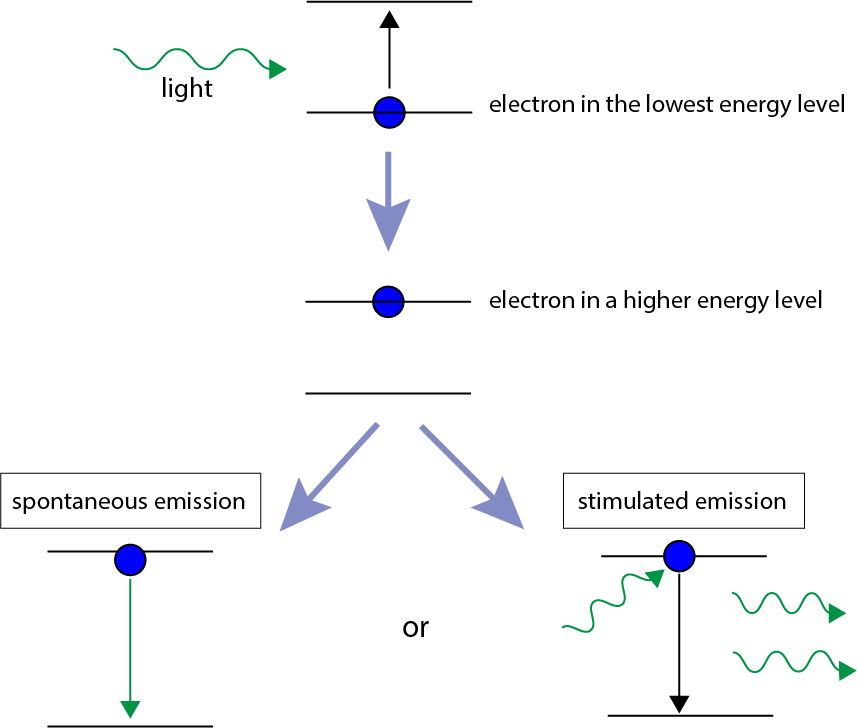
In order to exploit this mechanism of stimulated emission for the construction of a device that emits monochromatic, coherent radiation – a laser – there are three necessary components (Figure 4):
- An energy source for the initial input of large amounts of energy promoting many electrons in the active medium into higher energy levels; this could be an optical (flash lamp, or another laser), electrical or chemical source of energy.
- An active medium; a collection of atoms or molecules in the gas, liquid or solid phase. The active medium absorbs the energy from the excitation energy source and undergoes stimulated emission. The exact nature of the active medium determines the wavelength a particular laser emits.
- An optical cavity, consisting of two mirrors placed parallel to each other and allowing the stimulating photons to travel back and forth multiple times, amplifying the production of monochromatic and coherent radiation. One of the mirrors is constructed such that it permits controlled release of the amplified, focussed, directional laser beam from the optical cavity.
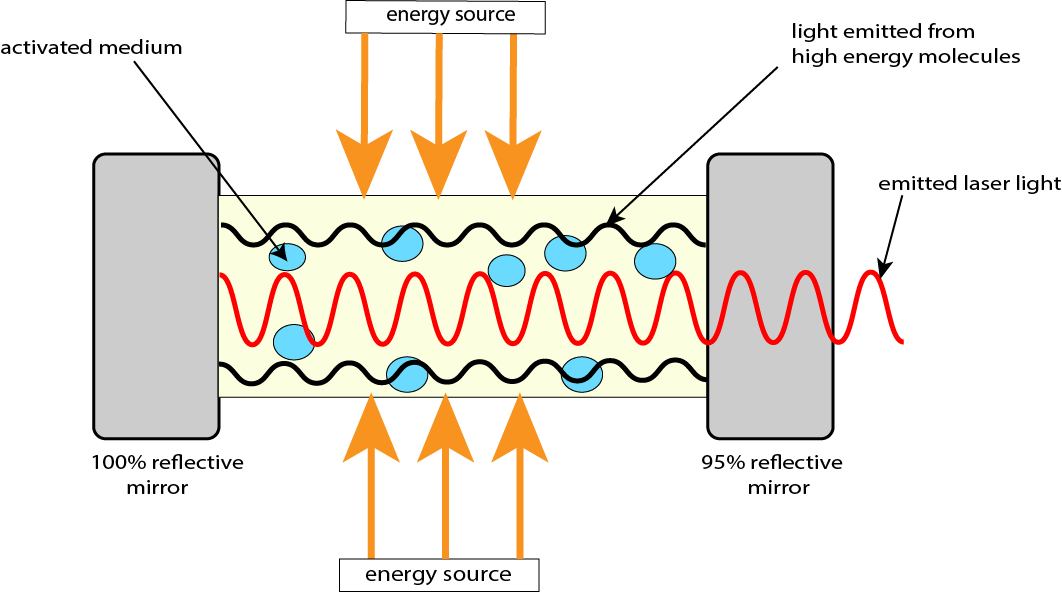
Over the years, lasers have been constructed and optimised with many different properties and applications in mind, including extremely narrow bandwidth of emitted electromagnetic radiation, extremely short pulses (crucial to study very fast processes in biological and chemical systems), extremely powerful pulses, covering a very wide range of frequencies with different lasers, creating directed laser beams that preserve coherence over incredibly long distances (important in space sciences). Lasers, therefore, find use across a wide range of applications – from the scanner of the supermarket cash point, to military purposes, industrial applications such as welding and cutting, to high-precision spectroscopy tools and medical applications.
Lasers in oral and maxillofacial surgery
In common with all other interactions of electromagnetic radiation with matter (including living biological systems), there are four main mechanisms how a laser beam interacts with matter: transmittance, absorbance, reflection and scattering (Figure 5).
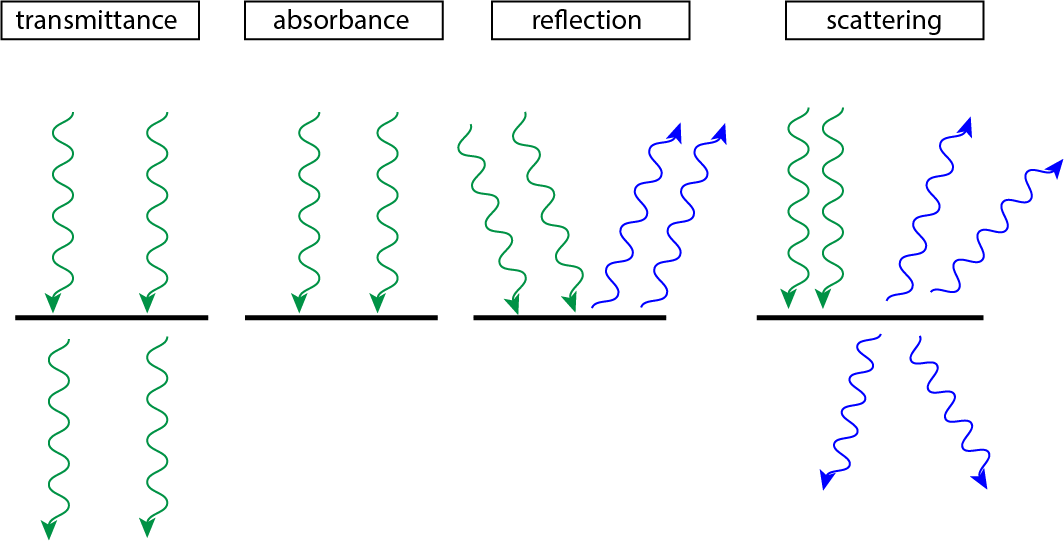
The most relevant interaction mode for surgical applications of lasers is absorbance, with some additional aspects of transmission also important for functionality.
Lasers used in surgical applications employ a small range (Figure 6) of the spectrum of electromagnetic radiation, approximately covering the range from infrared to ultraviolet electromagnetic radiation.
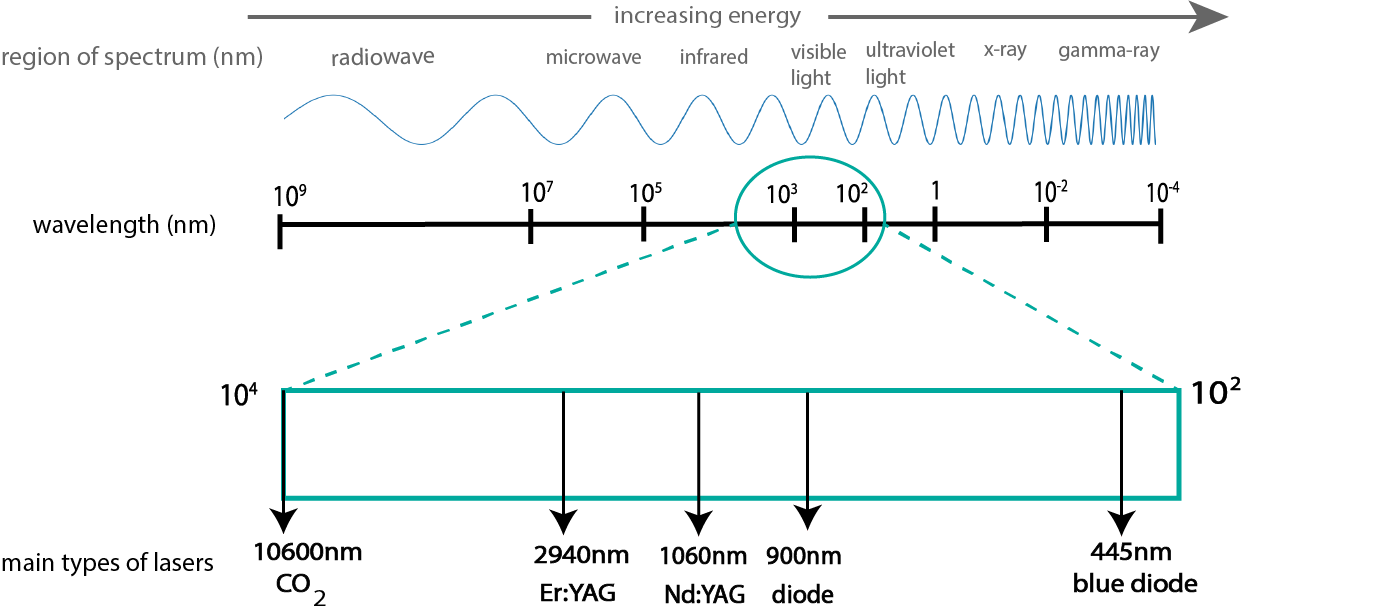
This frequency/wavelength range is suitable for a range of different surgical applications. When laser light is absorbed by biological material, there is a transfer of energy (for example, from light to heat). If selective absorption occurs for certain frequencies/wavelengths in selected tissue types, the amount of damage to surrounding tissues is minimised. Different specific lasers, with optimal properties for different surgical purposes are in use clinically. Figure 7 summarises the most important absorbance characteristics of the main targets (chromophores: water, melanin (a pigment in skin), haemoglobin (the component of blood carrying oxygen molecules around the body), together with the types of lasers with emission characteristics in the respective frequency/wavelength windows.
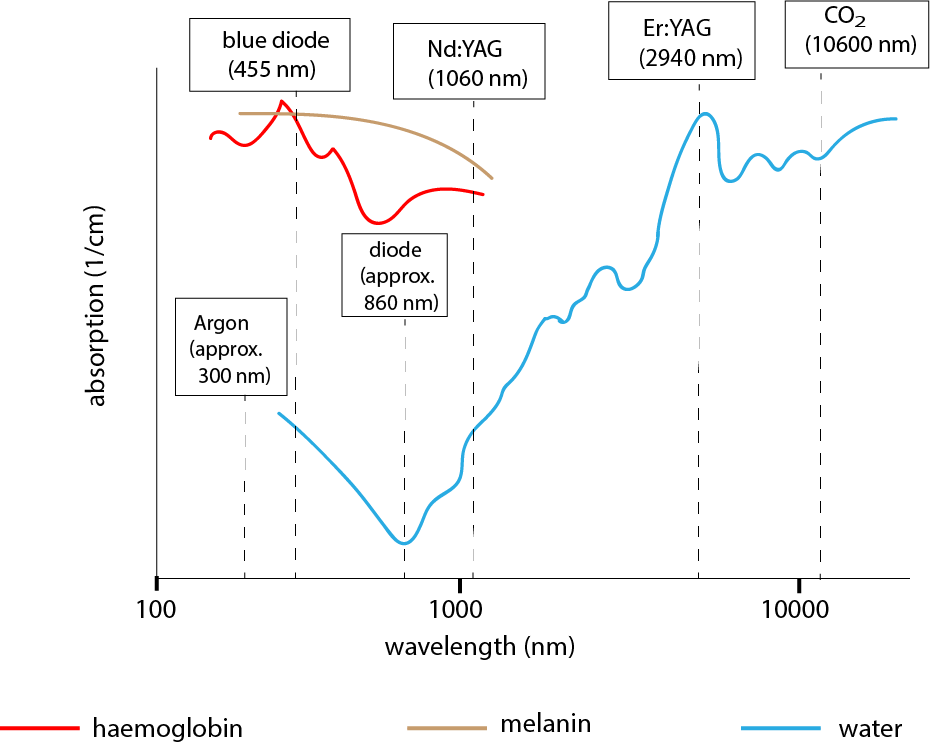
The main absorbance characteristics are:
- melanin – ultraviolet, visible and some regions of infrared radiation;
- haemoglobin – ultraviolet, and green and yellow components of visible light;
- water – strong absorption in the infrared and ultraviolet regions of radiation.
Absorption occurs through three main categories of interaction between tissues and laser photons:
- photo-thermal effects – conversion of radiation energy to heat (useful for coagulation and cutting of tissues);
- photo-chemical effects – inducing a chemical reaction in tissue (photodynamic therapy utilises this effect);
- photo-ablation – direct damage to biological structures by high-energy radiation effects without heating effects.
Another important factor to consider in surgical laser applications is thermal relaxation. Thermal relaxation is defined as the time required for the temperature of the absorbing chromophore (unit) to cool to half of its maximum temperature after the application of laser radiation. This relaxation rate is directly related to the size of the unit (faster cooling for smaller units than for bigger ones) and to the properties of surrounding tissues. In order to avoid thermal damage to surrounding tissues, the pulse duration of laser irradiation must be shorter than the thermal relaxation time.
By and large, advantages and disadvantages of lasers compared with other surgical techniques can be summarised as
- advantages – high precision for cutting soft tissues; reduced bleeding; sterilisation effect (‘sanitised surgical wound’);
- disadvantages – thermal damage to surrounding tissues; lack of haptic feedback on penetration depth during execution.
As summarised in Figure 7 (see above), the commonest lasers used in oral and maxillofacial surgery are CO2, Nd:YAG, Er:YAG, argon and (a variety of) diode lasers. These types of lasers are described below.
The active medium of a CO2 laser consists of gaseous CO2 (carbon dioxide), He (helium) and Ar (argon). The emitted light has a wavelength of 10,600 nm. Water is the only biological chromophore that absorbs radiation at 10,600 nm. The strong absorption by water molecules limits the penetration depth of this laser to a fraction of a mm. The main effect is photo-thermal: the energy absorbed by water molecules is converted to heat (when the temperature of the water in tissue reaches 100 ºC, water vapour forms and this volume expansion results in tissue fragmentation and, therefore, cutting. The CO2 laser is considered the surgical workhorse amongst the various lasers, its main application is for cutting and vaporisation (for example, in the removal of premalignant lesions of skin or mucosa) of soft tissues, in particular the tongue. The CO2 laser can be used in conjunction with a microscope in conventional (‘open’) oral and maxillofacial surgery but is also compatible with transoral robotic surgery approaches, TORS. Resection of tongue base and posterior pharyngeal malignancy using laser via TORS can obviate the more invasive approach of labiomandibulotomy (splitting of lip and mandible to gain access) in open surgery.
The active medium of a Er:YAG laser consists of an yttrium-aluminium garnet (YAG) crystal, doped with a small amount of erbium, Er. The emitted light has a wavelength of 2940 nm. This wavelength is strongly absorbed by water molecules as well as by hydroxyl apatite (the mineral component of bone), enabling the Er:YAG laser as a cutting tool for bone. The main effect of the Er:YAG laser on soft tissue is photo-thermal, but photo-mechanical (cracking of the bone matrix) effects dominate in hard tissue.
The active medium of a Nd:YAG laser consists of an yttrium-aluminium garnet (YAG) crystal, doped with a small amount of neodymium, Nd. The emitted light has a wavelength of 1060 nm. Both haemoglobin and melamin strongly absorb this wavelength of radiation, which is responsible for both the strong photo-thermal effect and the strong haemostatic (reducing bleeding) effect of the Nd:YAG laser at low power (ca. 30 W). Hence, this laser is predominantly used for its coagulation effects, but cutting can also be achieved by using it in contact mode with a carbon tip, or using a combination of a Nd:YAG with a CO2 laser. The Nd:YAG laser has a role in surgical interventions where there is a risk of serious bleeding from deficient blood coagulation, either by a range of diseases or related to anticoagulant.
The active medium of a diode laser consists of a semi-conductor, doped with gallium, Ga, or arsenic, As. The wavelength produced depends on the precise nature of the semi-conductor material used but is typically in the region 810 to 980 nm. This wavelength is strongly absorbed by melanin but poorly absorbed by water molecules. The main role of diode lasers is in achieving coagulation, applications in the treatment of peri-implantitis have been reported, quoting antimicrobial effects. Diode lasers with light emission in the visible red light region are used in photodynamic therapy . In maxillofacial surgery, there was a period when temporfin was the photosensitizing agent used in photodynamic therapy of specific cases of oral and oro-pharyngeal cancer but this application has stopped in the UK. after NICE found insufficient evidence to support the technique. However, photodynamic therapy is a technique, with different photosensitizing agents, that continues to be used on skin cancers .
The active medium of a blue diode laser can be a number of different semi-conductor materials; the active medium of the blue diode laser emitting a wavelength of 445 nm consists of a particular gallium-indium-nitride (GaInN) material. This particular diode laser is gaining popularity, as it is becoming increasingly economical and has some distinct advantages over other diode lasers operating in the infrared region of radiation. It features high absorbance in most soft tissues by melanin and haemoglobin, but poor absorption by water molecules, contributing to its effectiveness at very low powers. Excellent soft tissue healing has been reported after ablation and in contrast to lasers working in the ultraviolet region of radiation, the blue diode laser radiation has no adverse effects on DNA (or other) molecules in cells. The blue diode laser is a relatively recent addition to the surgical toolbox, its main advantages and disadvantages still need further investigations.
The active medium of an argon laser is slightly different in that it is made up of an ionised noble gas argon, Ar. The argon laser emits radiation in the range 250 to 520 nm (depending on the exact preparation conditions) which is strongly absorbed by haemoglobin and melamin. A major application area for this type of laser is in eye surgery (for example, repairing a ruptured retina), otherwise it is used in vascular surgery.
Diathermy and coblation
The working principles of diathermy and coblation, relying on an electrical current, revolve around the basic laws of physics relating current (the amount of moving charges (electrons) per time unit), voltage (the potential energy difference between two points in a circuit, the driving force for a current) and resistance (the tendency of a material to hinder the flow of charge) with each other.
Diathermy
The term diathermy refers to the cutting and coagulation of body tissue using a high frequency electrical current, resulting in tissue heating. An electric generator creates a voltage, the alternating current (AC) used is in the frequency range 100 kHz to 5 MHz (radiofrequency range) for electrosurgical applications. Using a frequency above 100 kHz is advantageous because neuromuscular stimulation ceases at this frequency; therefore, postoperative pain, swelling and spasms can be minimised.
When a current is supplied to tissues in the body, the tissues provide resistance and heat is produced locally (similar to the way a traditional light bulb works). The heat generated from this resistance results in localised boiling of intracellular fluid and this phenomenon enables the tissue to be cut.
Current density is also important and is related to resistance. Current density is the amount of current per unit area. Varying the current density changes the amount of heating (due to the relationship with resistance). Resistance is also proportional to size: the resistance increases as the area decreases. Surgical tools utilise this effect by having a small tip, which produces a large current density and large amounts of heat at the surgical site. Conversely, the return electrode in monopolar diathermy (see below) has a small current density because its surface area is large.
There are two main modes of diathermy, monopolar and bipolar.
In monopolar diathermy, the active electrode is at the surgical site but the return plate/ electrode is elsewhere on the body (usually around the thigh). The surgical tool introduces the electric current at the surgical site and the current passes through the body, which completes the circuit between the active electrode and return electrode (Figure 8). The Colorado needle is a variant of a monopolar diathermy instrument with a particularly sharp tip, designed for high precision cutting, dissecting and coagulation.
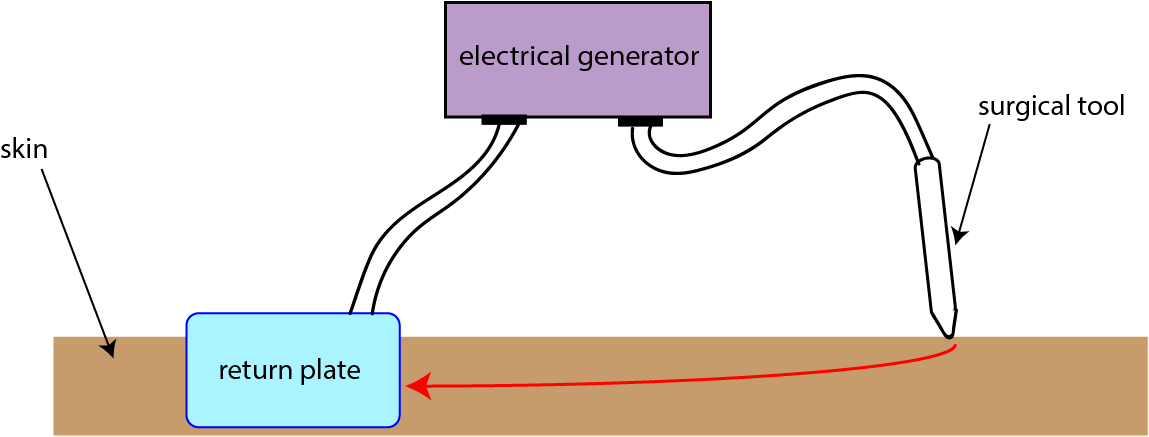
In bipolar diathermy the patient's body is not used to complete the circuit, the electrical current is localised at the surgical site. The current applied during bipolar diathermy only passes through tissue between the two arms of the forceps (surgical tool) (Figure 9).
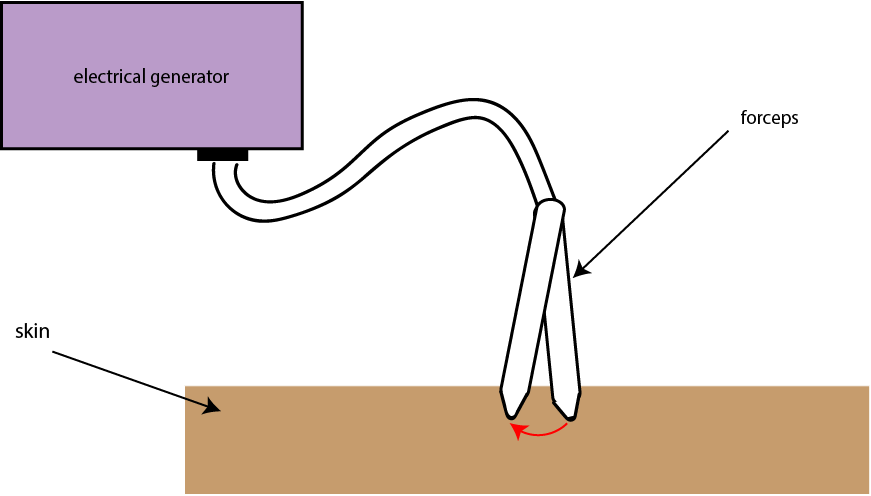
Figure 10 depicts the actual surgical instruments used in mono-and bipolar diathermy.
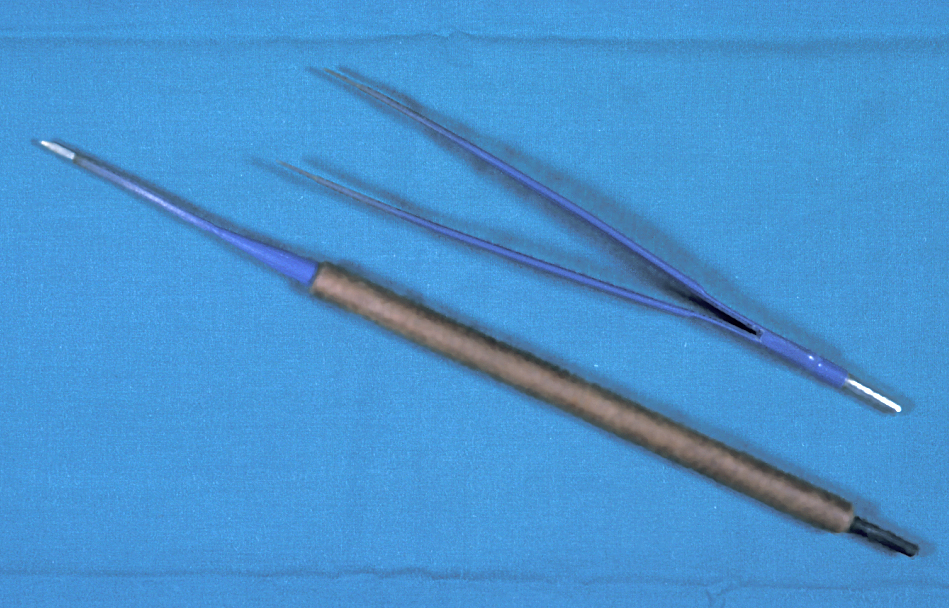
The main use of monopolar diathermy is cutting of tissues, for example in tongue resections. Bipolar diathermy is mainly used as a forceps, to coagulate small vessels, particularly when flaps are being used in reconstructive surgery. The so-called harmonic scalpel is a non-electrical alternative for simultaneous cutting and coagulation; its working principle is to apply ultrasonic vibrations.
Coblation (controlled ablation)
This technique is related to diathermy in that both techniques use alternating currents to achieve the desired effects. Unlike diathermy, coblation is not a heat-driven process. Instead of heating the tissue by a high-frequency alternating electrical current delivered directly to tissue as in diathermy, in coblation the radiofrequency is delivered to a conductive medium (for example, an aqueous solution of sodium chloride) surrounding the active electrode. This creates a medium known as a low-temperature plasma. Reaction of the plasma with surrounding water molecules creates highly reactive species (radicals, such as OH, O and H species with unpaired electrons). These short-lived reactive chemical species can break / disrupt chemical bonds in nearby tissues, with very little or no bleeding but with less-than-perfect control of the tissue removal achieved. Coblation is sometimes used in parotidectomies (removal of the parotid gland (one of the major salivary glands)) or in neck dissections.
Cryotherapy
Cryotherapy takes us to the extreme opposite of the temperature range by exploiting the very low temperature of liquid nitrogen, N2 (-196 ºC).
Application of liquid N2 leads to tissue destruction by direct and indirect routes. Direct routes involve the formation of ice crystals in fluid outside cells and within the cells, leading to localised cell membrane damage, inhibition of enzymes and protein damage, and ultimately to the destruction of the cells. All of these direct routes cause the cells exposed to liquid N2 to swell and eventually to rupture because of the presence of water in cells. The underlying mechanism is the unique property of water, known as the water anomaly. Water is most dense at temperatures a little higher than its melting point of 0 °C. On further cooling (and freezing), its density reduces and, in consequence the volume expands in frozen water. This effect ensures the survival of fish in frozen lakes (as there will nearly always be liquid, albeit cold, water at the bottom of the lake) and explains the explosion of a bottle of beer (or other beverages) that one placed in the freezer for a quick cooling but forgot to remove after a short time. In cryotherapy, the water molecules in the cells initially contract on cooling, further cooling lets the ice crystals expand, leading to rupture of the cell (on perfect analogy to the forgotten beer bottle in the freezer). Indirect mechanisms of tissue destruction by cryotherapy include a stimulated immune response and restricted blood flow in the treated area. All of these mechanisms can lead to tissue necrosis.
Cryotherapy is mostly used for the removal of superficial (skin and mucosa) lesions, small vascular lesions, mucoceles (a form of cyst), or papillomata. Another application of cryotherapy is in the treatment of refractory and neuropathic pain. If a clear diagnosis (for example, by applying a nerve block) can be made and the nerve identified, applying liquid N2 to the nerve via a medium (usually petroleum jelly) and repeating a cycle of freezing and thawing a few times, will damage the nerve enough to typically lead to several months of non-function of the nerve, with the beneficial side-effect that the nerve will also be unable to transfer pain signals. It should be noted, though, that the evidence for the treatment of neuropathic pain by cryotherapy is slim and in part controversial.