Drug design
In the workings of biological systems, nature has adopted the ‘form follows function’ principle in nearly all cases where we currently have a detailed understanding of biochemical and metabolic processes. This accounts for the many highly specific roles that messenger molecules, antibodies, receptors, and so on play in the normal functions of living systems. In addition, the action mechanisms of medicinal and recreational drugs follow the same principle. In the past, many medicinal drugs were typically discovered in a laboratory-based, work-intense trial & error approach of synthesizing very large numbers of variations of a particular drug candidate molecule, and checking all these chemicals for their wanted and unwanted properties in a first step.
The basic tools in modern drug design
The second half of the 20th century has seen much progress in the
- development of biochemical laboratory procedures (such as isolating and crystallising proteins, or isotope-labelling strategies for subsequent investigations of structure and function of proteins);
- structure-elucidation methods suitable for the investigation of the structure of large bio-molecules (specialised X-ray diffraction techniques, special microscopy methods and spectroscopy tools, in particular solution- and solid-state nuclear magnetic resonance (NMR) spectroscopy);
- massively enhanced computing hardware / computing power as well as the emergence of powerful software for the analysis and manipulation of structural data of large biomolecules, including visualisation tools.
Taking all these advanced technologies together is key to state of the art approaches to a far more rational design of promising drug molecules. Drug design depends on the knowledge of the target structure / receptor and their role(s) in a biological system. Equipped with this knowledge, it is then possible to design, with the help of computer algorithms, promising molecular candidates for specific medicinal tasks, including constraints on their predicted properties, for example restricting adverse effects. Only the most promising candidates from such an electronic library of molecules will need to be synthesized in the laboratory for further pre-clinical testing – and for a whole raft of reasons, only very few of these candidates will actually ever become medicinal drugs in clinical use after many years of further testing and different stages of clinical trials.
Re-designing fentanyl
One class of medicinal drugs where drug design is a promising approach to improve on the existing pool of drugs is the vast group of opioid analgesics Opioids are highly potent agents to counteract pain. The effects are achieved by the docking of opioids at a range of different opioid receptors, some of which are located in the central nervous system (brain). Interactions with opioid receptor sites in the brain are responsible for the adverse effects of opioids, including potential for addiction, sedation and respiratory depression.
Fentanyl (Figure 1) is a powerful opioid analgesic, but carries a substantial risk of these adverse effects. The objective of a recent study to re-design fentanyl was to maintain its powerful analgesic action whilst dropping the adverse effects.
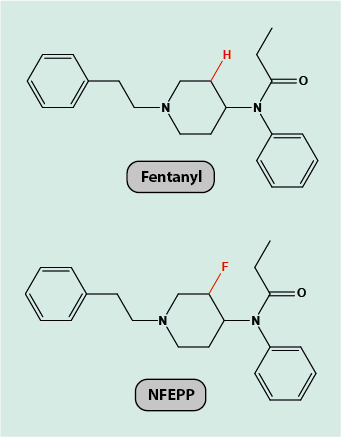
The structures of fentanyl and its receptor site are known. This information permits the deduction of a mechanism of action of the drug, by chemically interacting in specific ways with the receptor site. It is further well researched that painful inflammation of tissues goes along with the afflicted tissue being more acidic than normal tissue (in fact, locally enhanced levels of acidity in inflamed tissue can be a reason for failure of local anaesthesia).
Accordingly, the drug design task for finding a re-designed fentanyl derivative can be summarised as follows: find a modification of fentanyl that preserves its powerful analgesic action but only binds to opioid receptor sites in environments with enhanced acidity. This can be achieved by simulating an inflammatory situation and by varying the acidity levels in the virtual environment (which would be impossible to do in a real laboratory experiment), and varying the exact molecular drug composition for optimum binding. At least for the management of severe pain caused by inflammation, this would be an attractive modification to a powerful opioid (but would not cover other analgesia applications). Figure 1 shows the result of searching for a fentanyl derivative with the specified properties, called NFEPP. The modification is really minor, with only two hydrogen atoms being replaced by fluorine atoms.
Subsequently, NFEPP has been synthesized in the laboratory to be compared with the actions of fentanyl. NFEPP was initially tested on some cell lines to demonstrate that indeed it is preferentially taken up in more acidic environments. In the usual following step in the procedures of characterising a promising drug candidate, the analgesic properties of NFEPP have been tested and confirmed in rats. Additional experiments on rats have also demonstrated that NFEPP has no action on central nervous opioid receptors but solely docks on the receptors in inflamed tissue and/or sites of injury in general where the pain signals originate.
NFEPP thus has taken a first step from the computer to the laboratory. From there, it will be many years until it will be known if NFEPP (or further optimised versions of it) becomes a clinically useful drug.
The case study of NFEPP not only illustrates some general principles in the design of new and/or improved drugs. It also gives food for thought in terms of analgesics in general. NSAIDs such as ibuprofen are over-the-counter analgesic drugs, widely used and with a range of adverse effects, although they do not carry an addiction risk. For example, NSAIDs can cause serious problems such as gastric ulcers and bleeds. More recently it has been found that NSAIDs also have substantive adverse effects on the cardiovascular system. Similar drug design & optimisation philosophies and techniques could be applied for finding improved NSAIDs with fewer and less severe adverse effects.