Hard tissue necrosis
Contents
Below we investigate in a little more detail:
- how the bone remodelling process works normally to maintain a healthy bone structure throughout life;
- how high-energy radiation (radiotherapy) can interrupt and impair this process and lead to hard tissue necrosis;
- how some medications can interrupt and impair this process and lead to hard tissue necrosis.
The discussion is a brief account of the current ideas about some of the bone remodelling processes. It outlines a work in progress in many different regards. In particular, some effects of high-energy radiation and bioavailability of bisphosphonates and other antiresorptive medications (see below) on healthy bone tissue are only incompletely understood. Better understanding of the physiology and pathophysiology of bone remodelling is vital in order to be able to design rational treatment schemes, in particular where medications or exposure to high-energy radiation are the cause of bone necrosis.
The bone remodelling process
The general macroscopic picture
Bones have structural and motor functions, as well as being a mineral reservoir (Ca2+ ions). Remodelling of bones is a life-long renewal process, necessary to retain bone strength and to maintain healthy Ca2+ ion levels in plasma. It is estimated that approximately 5 to 10 % of the skeleton of an adult is renewed over the period of a year. There are two types of bone tissue. Overall cortical bone makes up approximately 80 % of the skeleton. It is a heavily mineralised, mechanically strong bone tissue and its main task is to maintain structure and to provide protection of delicate tissues. Cancellous (‘spongy’) bone makes up the remaining approximately 20 % of the skeletal bone. Cancellous bone is much softer than cortical bone and because of its spongy, porous structure has a large surface area that allows for its high metabolic activity. The distribution of cortical and cancellous bone tissue is not uniform, it varies with the roles of different bones in the body. The mandible, like the ilium (part of the hip bone) and tibia (shinbone), has a relatively large amount of cancellous bone in adulthood, whereas the maxilla comprises thin sheets of cortical bone. The zygoma (cheekbone) and calvarium (skullcap) are essentially dense cortical bone with a thin layer of cancellous bone like the filling in a sandwich.
The bone tissue itself is a masterpiece of tissue / composite material engineering – it is a light-weight, yet mechanically strong material. The bone mineral component (calcium hydroxyl apatite (Ca10(PO4)6(OH)2), a phosphate mineral with an intricate three-dimensional structure) provides stiffness. The organic component, the bone matrix, is mostly made up from partially ordered collagen fibres (with further proteins hosted in the tissue) and makes bones tough and resistant to fracture. A particular interwoven and partially aligned architecture of mineral and organic components in this composite material bone achieves these amazing mechanical properties. Bone conditions that lead to an overall distorted architecture of bone tissue (for example, Paget’s disease) from chaotic remodelling processes thus weaken the bone.
The general microscopic picture
The two main players in the bone remodelling process, in addition to extensive signalling pathways for coordination (see below), are osteoclasts and osteoblasts. These are specialist types of cells, which together execute the bone remodelling process in a highly integrated and coordinated manner. Osteoclasts are responsible for the resorption of spent / old bone tissue, osteoblasts are the cells that lay down new bone tissue. It is important for this process to be well orchestrated, balanced and to proceed in such a way that the resulting overall volume and strength / structure of bone is not altered, and that the renewal process itself does not unduly weaken the bone temporarily.
The renewal process is systemically triggered by parathyroid hormone (PTH) when Ca2+ ion levels are low, osteoclasts are then generally activated. Many different hormones play a role in bone remodelling, amongst these, oestrogen has a major influence. Oestrogen reduces osteoclast activity, promotes osteoclast apoptosis as well as osteoblast activity. These activities typically are in balance in women before menopause. When oestrogen levels fall steeply in older women after menopause, an imbalance of the bone remodelling process results. That could be within the boundaries of the normal ageing process. However, this can be problematic if the rate of remodelling activation and bone resorption increases significantly but there is not an equivalent balanced degree of quality-bone rebuilding. Altogether this cycle then leads to a growing imbalance of bone remodelling over time (osteoporosis) and results in significantly weakened bones. It is this cycle that some widely prescribed medications for the treatment of osteoporosis aim to interrupt (see below).
Locally, micro-damage and/or mechanical load to the bone are thought to be sensed by osteocytes (osteocytes are a type of cells to which osteoblasts revert once their bone-building task is completed and they get embedded in the newly formed bone tissue (osteoid) / bone matrix; osteocytes are the most common type of cells in bone) which then release signalling to activate the local remodelling process by activation of osteoclasts.
The local chronology of events in bone remodelling
Figure 1 gives a simplified cartoon version of the local events in the bone remodelling process.
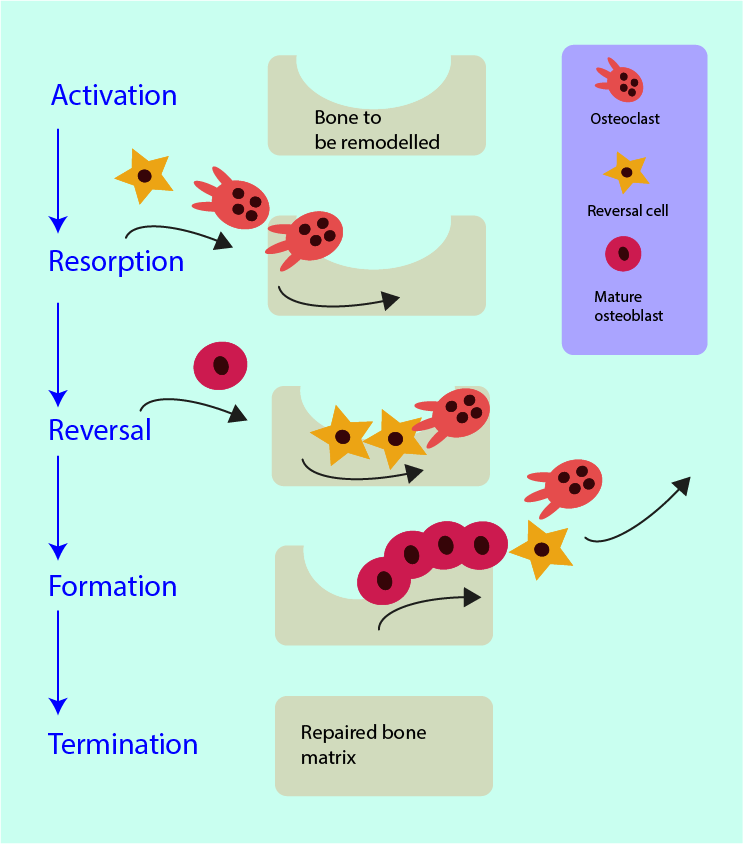
The bone remodelling process can be activated locally and/or systemically. The local activation signal in an area of a bone lesion stems from the apoptosis of osteocytes which activates osteoclasts. The systemic triggers are hormonally controlled feedback loops. Once the process is activated, locally or systemically, a temporary cluster of the active cellular units assembles. These basic multicellular units (BMU) form a work unit with specific allocated tasks for all its members. It is a highly spatially and temporally organised unit which needs multiple signalling and feedback paths for it to function properly. The ‘get ready’ signalling protein (RANKL) after activation is issued by osteoblast cells. RANKL binds to the RANK receptor of osteoclast precursor cells and activates those into fully developed mature osteoclasts that are subsequently recruited to the remodelling site. There is an antagonist (opposite player) protein to counteract the effects of RANKL: osteoprotegerin (OPG) is also released by osteoblast cells, it is regulated by the hormone oestrogen and reduces the formation of mature osteoclasts. The RANKL/RANK/OPG interplay is vital to keep bone formation and resorption in balance.
The working front end of the BMU in the resorption phase of bone remodelling are the mature osteoclasts. Osteoclasts are large multinuclear cells with ruffled borders. This helps to attach to the surface of the bone defect and seal it. Next the osteoclasts release enzymes to digest the organic bone matter (mostly collagen fibres) and acid to dissolve the inorganic bone mineral. The osteoclast action creates space for new bone tissue but leaves some debris behind, mostly consisting of undigested collagen fibres and fragments.
This calls into action the so-called reversal cells which follow the paths of the osteoblasts and prepare the bone surface for the deposition of new bone material. Reversal cells remove the remaining debris and cover the surface. The exact nature (phenotype) of reversal cells is a matter of debate but it has been established recently that these cells belong to the lineage of osteoblast cells and thus are probably not just a specialised type of macrophage (cell that specialises in engulfing and digesting material). There may be differences in the reversal cells and reversal phase of bone remodelling in different mammals, hence experimental findings across species need to be interpreted cautiously. The final stage of the reversal phase is for the reversal cells to transmit and receive signalling to move on to the next stage of the process.
The formation phase of bone remodelling directs osteoblasts to the sites of bone resorption where they facilitate the formation of new bone tissue. The biochemical signalling pathways and exact mechanisms of this crucial stage of the bone remodelling process remain controversial.
Once osteoblasts have arrived at the remodelling site, these cells produce molecules that are the starting materials for the formation of new bone tissue. Primarily collagen fibres are deposited but also further proteins and lipids. This forms a kind of template (the osteoid) onto which the bone mineral components (calcium hydroxyl apatite (Ca10(PO4)6(OH)2) are deposited. This mineralisation process starts about two weeks after the initial deposit of osteoid by the osteoblasts, it continues for about six months and concludes in a late phase of secondary mineralisation and consolidation over two to three years. The exact mechanism of this process is not yet fully understood, it continues long after the osteoblasts and reversal cells have left the scene.
Once the missing volume of bone has been replaced, the process is locally terminated. It is thought that osteocytes play a signalling role at this stage but this hypothesis needs further investigations. Once the new bone tissue is mineralised, the osteoblasts either undergo apoptosis (programmed, orderly cell death), or restructure and become bone-lining cells or osteocytes embedded in the mineralised bone matrix.
We have taken a fair number of short cuts in this description. The bone remodelling process is more intricate with many more regulation, feedback and control cycles involved than our brief description implies. There are also several currently unanswered fundamental questions about the biochemistry and physiology of the process. For example, the roles of immune cells in general and inflammatory messaging are not well understood but are likely of clinical importance in the treatment of bone conditions.
Interference of high-energy radiation with the bone remodelling process
The destructive effects of high-energy radiation on healthy bone tissue were first described nearly 100 years ago (‘radiation osteitis’), yet to this day the understanding of the underlying pathophysiology remains incomplete. One intriguing question awaiting a comprehensive answer is: why is the mandible (lower jaw bone) so particularly vulnerable to radiation damage resulting in ORN? A relatively high bone turnover rate of the mandible alone does not appear to offer an exhaustive explanation. However, a high bone turnover rate combined with a particularly high risk of infections (for a variety of reasons; see below) and a reduced vascular supply in comparison with the maxilla (upper jaw) would seem a logical starting point for initial explanations.
A short history of the hypotheses about the pathophysiology of osteoradionecrosis (ORN) includes the following views:
- An established view of osteoradionecrosis (ORN) over several decades describes the condition as the result of the combined effects of hypoxic, hypovascular and hypocellular tissue from radiation damage, leading to breakdown in the form of bone necrosis; this hypothesis does not explicitly consider infection as an important contributing factor.
- Vascular damage, in particular to the inferior alveolar artery (which runs in the mandibular (lower jaw) canal and supplies the mandible and lower molar teeth) has been highlighted as a weak link.
- It was repeatedly pointed out that high-energy radiation causes irreparable damage to osteoclasts (see above), which leads to dysregulation of the bone remodelling processes and over time causes bone tissue breakdown. This hypothesis emphasizes some of the similarities of ORN with osteonecrosis caused by some medications (MRONJ; see below).
- The role of (bacterial and fungal) infections in the development of ORN has been widely discussed, highlighted and demonstrated in several studies; it continues to be confirmed.
- The most recent thinking about the pathophysiology of ORN ascribes its development to a fibroatrophic mechanism (the simultaneous occurrence of fibrosis (scarring) and atrophy (wasting) in irradiated tissue). It essentially interprets ORN as the catastrophic long-term result of the body’s attempts to repair radiation damage. The mechanism includes the effects of free radicals, inflammation, microvascular thrombosis and dysfunction of endothelial (lining) cells, leading to fibrosis and atrophy by preventing a normal bone remodelling process, before eventually ORN is established.
The mechanism is thought to process in three stages over several years: i) an initial acute inflammatory phase (a pre-fibrotic phase) which is mainly concerned with endothelial cells; ii) a constitutive (chaotic) phase which is mainly characterised by increasingly abnormal activity of fibroblasts and loss of organisation of extracellular tissue; iii) a late fibro-atrophic phase with repeated attempts of tissue remodelling with the formation of fragile tissues which are vulnerable to injury and repeated inflammation from even very minor insults.
This proposed mechanism bears some similarities with the traditional ORN model (hypoxia, hypovascularity, hypocellularity; see above) in that it does not emphasize the role of infections.
The main weakness of this fibroatrophic-mechanism hypothesis is that it was actually developed to explain long term soft tissue necrosis that occurs, for example, after radiotherapy to treat breast cancer. There are clearly differences in the wound healing processes of hard and soft tissues, as well as in the regular remodelling and renewal processes of soft and hard tissues. It has so far not been convincingly explained how a soft-tissue based model can be seamlessly applied to hard tissue (cellular and molecular biology studies of microscopic events in the development of necrosis, quoted as supporting this model of ORN development, focus on the study of soft tissues).
Recent histological investigations of resection specimens of mandibular ORN reveal that necrosis of cortical bone, especially near the inferior border of the mandible, is more common than necrosis of cancellous bone. This finding has been tentatively ascribed to a decrease in or lack of periosteal blood supply.
It would be clearly highly desirable to arrive at a more robust idea about the pathophysiology of ORN in order to enable better, rational treatment schemes for this condition.
Interference of medications with the bone remodelling process
Recalling the multiple steps and multiple regulation cycles and signalling pathways of the bone remodelling process, it is obvious that there will be as many opportunities for the treatment of bone diseases by medications as there are opportunities for things to go wrong and for unintended consequences to develop. The list of such medicinal drugs in use (or being trialled) is long and growing. In addition, some medicinal drugs that are used for other treatment purposes also have a profound effect on bone remodelling.
Bisphosphonates
The most widely used class of medicinal drugs for the treatment of osteoporosis and bone metastases is the group of so-called bisphosphonates (commonly abbreviated as BP). This class of drugs shares a common motive (Figure 2) of two phosphonate groups linked by a -CR1R2- unit.
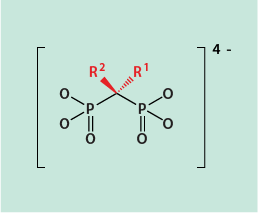
Depending on the chemical nature of the substituents R1, R2 in the molecule, the potency of bisphosphonates as antiresorptive agents varies widely and covers 4 orders of magnitude of potency. MRONJ from BP treatment currently is considered the most common form of osteonecrosis of the jaw (as a result of the very large number of people on long term BP treatment).
Bisphosphonates tend to bind strongly to the surface of bone (where the uptake is preferential and where they remain for a very long time) in remodelling sites, chelate Ca2+ ions, prevent osteoclast formation, hinder osteoclast function and promote osteoclast apoptosis in several ways, mainly by BP’s being engulfed by osteoclasts. The altered osteoclast profiles in MRONJ differ slightly from those in ORN.
Altogether the main BP effect will thus be a reduction in the resorption phase of the bone remodelling process and this should be expected to contribute toward strengthened bones. The list of actions of BP’s on osteoclasts gives a hint why tooth extractions are a common trigger event for MRONJ under BP treatment, significantly more often than for ORN (see above). The extraction socket is not only vulnerable to infection, it is also an exposed remodelling site. There is some evidence that the function of osteocytes (signalling of mechanical load status) and osteoblasts (altered mineralisation) is also directly affected by BP’s. In the longer term the presence of BP molecules embedded in the bone structure leads to changes in the mechanical properties of bone by disrupting the normal bone architecture by patchy MRONJ lesions (which has been tentatively ascribed to a local immunosuppressive effect of BP molecules). This is in slight contrast to histopathology findings in ORN where bone lesions tend to be more homogeneous and necrosis more extensive. Periosteal viability tends to be significantly higher in MRONJ than in ORN (with consequences for treatment options, where conservative surgical approaches for MRONJ are more successful than for ORN).
Denusomab
Denusomab is a human monoclonal antibody, that is an antibody designed to bind to just one type of receptor. Denusomab competes with RANKL (see above) for binding to the RANK receptor, with great affinity for RANK. Accordingly, denusomab inhibits bone resorption by inhibiting the development of mature osteoclasts from precursor cells. Other than bisphosphonates, denusomab does not alter the biomechanical properties of bone. It also has a shorter life span in the body than bisphosphonates, its effects on bone remodelling decrease significantly approximately 6 months after treatment cessation.
Other agents interacting with the bone remodelling process
During the resorption phase of the bone remodelling process, mature osteoclasts secret a lytic enzyme (cathepsin K) for the dissolution of collagen fibres. This role was identified as another promising target for antiresorptive drugs. Several inhibitors of cathepsin K have been developed, only one of these (odanacatib) has so far progressed to clinical trials for the treatment of postmenopausal osteoporosis. Despite promising effects on bone strength, these trials have not led to further commercial development of the inhibitor as trials revealed increased stroke risk.
Sclerostin is a protein that is produced by osteocytes and forms part of the osteocytes’ self-regulation / feedback loops; its main effect is a reduction of activity in the bone formation phase of the bone remodelling process (it is a counterplayer to PTH’s effects on bone remodelling). Sclerostin is specific to bone metabolism, which makes it an attractive target to counteract with an antibody, aiming to increase bone formation. Romosozumab is such an antibody currently undergoing clinical trials (2017), several other inhibitors of sclerostin are being developed.
Teriparatide is a protein closely related to the parathyroid hormone (PTH). It increases osteoblast activity and thus enhances bone formation. It is occasionally used to treat high-risk osteoporosis and to enhance the healing of fractures but is contraindicated for people at risk of developing osteosarcoma.
We mention some of these uncommon and/or newly developed medicinal agents to provide more context and an outlook to further developments – which may or may not present further ONJ challenges in the future. In addition, these selected cases illustrate the logic behind the common choice of antiresorptive drugs for the treatment of osteoporosis in particular. They are the lesser evil: reducing the resorption of bone, as opposed to increasing the formation of bone, is the less risky strategy especially for long term treatments. Exploiting general growth factors as systemic pharmaceuticals, or uninhibiting growth mechanisms in other ways carries an inherent risk of encouraging unintended malignant growth.
Antiangiogenics are a group of medicinal drugs which hinder blood-vessel growth in various different ways. They are a (controversial) type of medication used in the treatment of malignant tumours, with the idea that preventing the growth of new blood vessels to supply tumour tissue will ‘starve’ the tumour.
The most common of these agents are bevacizumab, sunitinib and sorafenib. The different substances block different growth factors for vascular (blood vessel) growth. These medications are administered systemically, hence their action also affects healthy tissue and blood vessels. The mandible is vulnerable to impairment of its vascular supply and MRONJ following treatment with antiangiogenics has been reported.