Stem cells
Contents
Stem cells show great potential for use in the treatment of many conditions, including a range of oral and maxillofacial problems. Using their natural ability to divide and differentiate into multiple cell types, stem cells could be manipulated to revolutionise tissue engineering, the repair or replacement of tissue. They could help replace both hard tissues (bone, cartilage) and soft tissues (skin, muscle, salivary glands), enabling use in procedures such as bone reconstruction, skin replacement following burns and other defects, cleft lip and palate repair, replacement of teeth, or xerostomia treatment.
As with all treatment modalities, the use of stem cells is not without risk. For example, in the treatment of some haematological malignancies, stem cells transplanted from other individuals have been found to sometimes reject their host environment, attacking the host in what is known as graft versus host disease (see below). Perhaps counterintuitively, stem cells are also thought to directly promote progression of some pathological conditions. For example, cancer stem cells use the replicative and tissue-forming properties of stem cells to promote cancer development (see below).
Below we look at the various sources of adult stem cells and their potential uses in oral and maxillofacial surgery, as well as the problems they can cause through rejection, and stem-cell involvement in disease development & progression of malignancies.
Sources of stem cells
Stem cells can be found naturally in early human development (in embryos or pre-embryo structures) or in adult tissues. Stem cells can also be formed by manipulating adult cells (induced pluripotent stem cells). We focus on adult stem cells. Adult stem cells are located across the whole body, from bone marrow to skin, fat, liver, heart, brain, teeth and muscle tissues. Figure 1 provides an overview of just a small proportion of the many sources and types of stem cells found in an adult body.
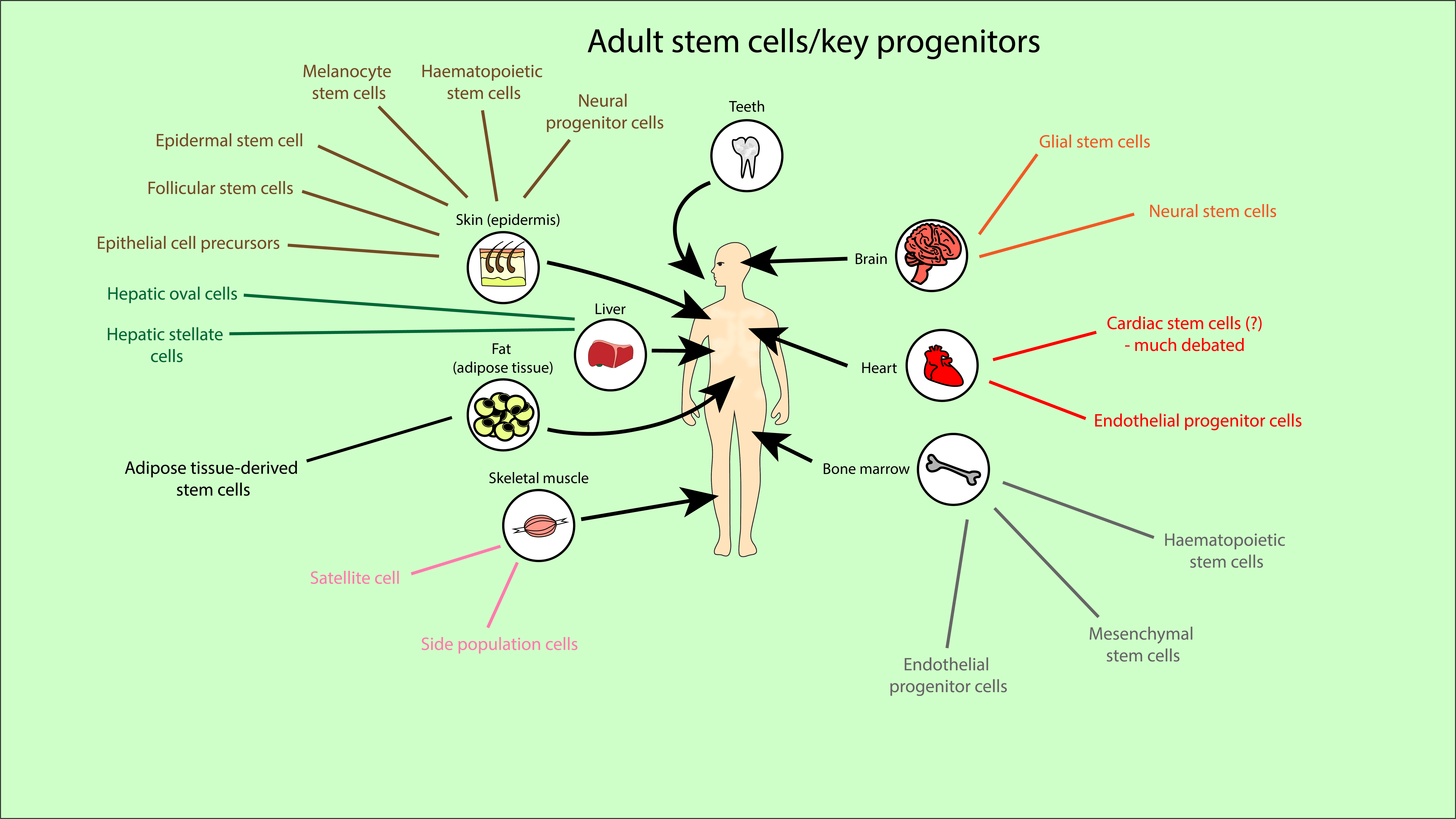
Mesenchymal stem cells are a subset of stem cells which are a key player in regenerative-medicine research. They are multipotent stem cells, with a tendency to differentiate into bone, muscle, fat and cartilage cells, but also have the potential to develop into a wider array of cells including neural cells. This broadens their medicinal potential to treatment of neurodegenerative diseases such as Parkinson’s disease and Alzheimer’s disease.
Mesenchymal stem cells have several key properties which suggest medicinal potential:
- multipotency;
- ability to self-renew;
- relatively easy to expand in the correct conditions (culturing the cells);
- ‘homing’ to sites of injury – mesenchymal stem cells can migrate to sites of injury (although this ability has limitations);
- immune modulatory properties – could prove useful in the treatment of autoimmune diseases or reduction of inflammation;
- a highly stable genome (so unlikely to express unexpected genes, clear differentiation pathway, helps safety).
Mesenchymal stem cells were first discovered in the bone marrow (Figure 1), but mesenchymal stem cell-like cells have been isolated from all over the body. For example, they have been found in both foetal tissues (such as amniotic fluid/membrane, the placenta, foetal membrane and limb buds) and adult tissues (such as fat tissue, dental tissues, skin and salivary glands). These alternatives retain key mesenchymal-like properties such as multipotency and the ability to self-renew, whilst not always requiring a difficult extraction process like that from bone marrow. These alternatives also have different tendencies towards forming specific cell types, suggesting they could not only be a replacement, but a more effective source of stem cells for certain treatments.
Numerous mesenchymal-like stem cell populations have been located in the mouth, and are being investigated for uses in oral and maxillofacial surgery. These stem cells are thought to have great potential here due to their mesenchymal-like properties (multipotency, self-renewal) and tendency to differentiate into cells which form bone (osteogenic cells) and teeth (odontogenic cells). Various different sources have been discovered, including gingival (gum) and dental structures. Common dental sources include (Figure 2):
- the dental pulp inside teeth (dental pulp stem cells);
- the ligaments surrounding teeth (periodontal ligament stem cells);
- exfoliated ‘baby teeth’ (stem cells from exfoliated deciduous teeth are a completely non-invasively accessible source);
- tissue present during dental root development (stem cells from apical papilla);
- stem cells involved in early tooth development (dental follicle progenitor cells);
- cysts which can form at the base of roots (human periapical cyst mesenchymal stem cells).
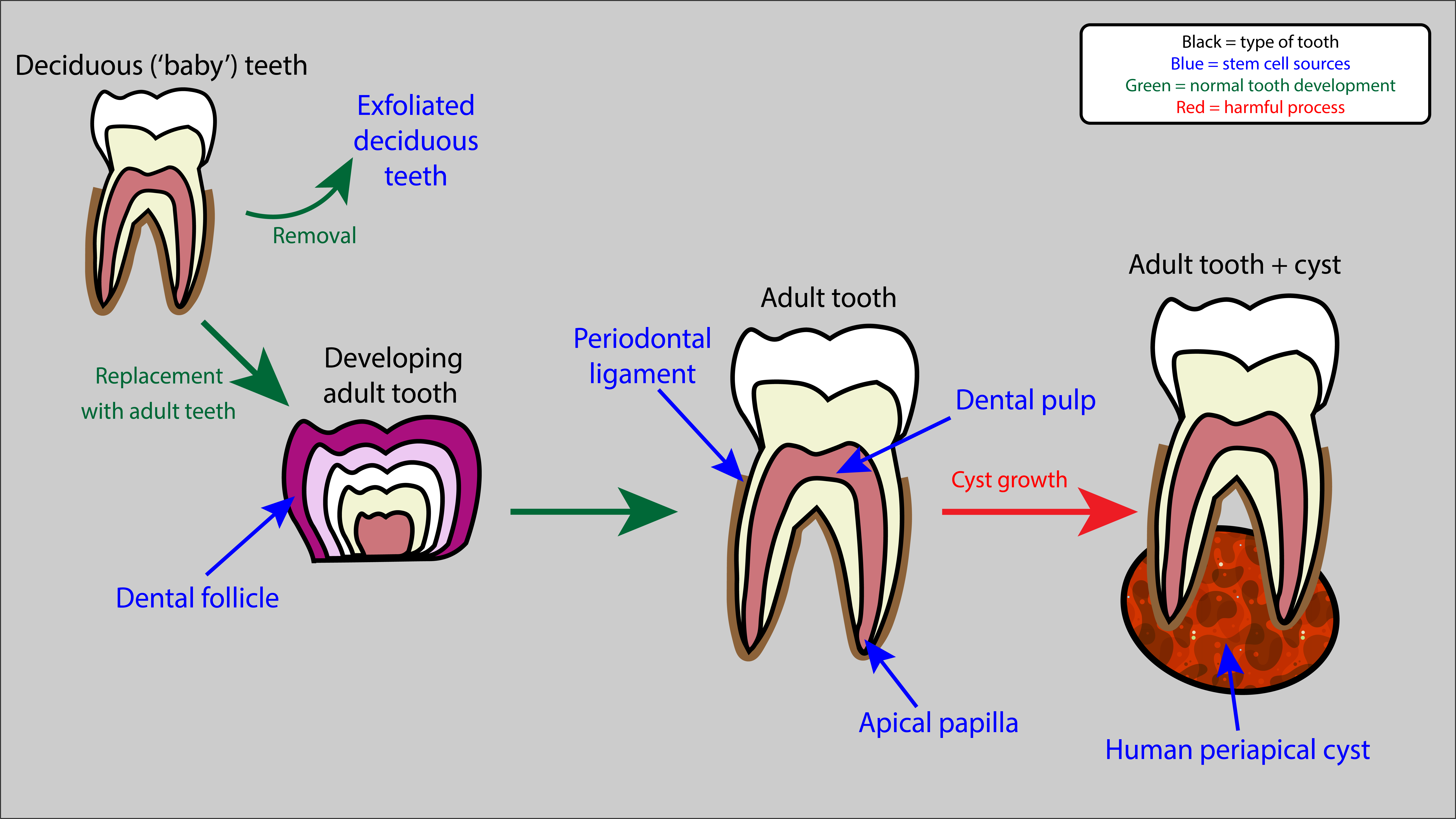
Dental stem cells are much more easily extracted than those in bone marrow, often from sources which would be extracted (or exfoliated) anyway and otherwise go to waste (cysts or deciduous teeth). Once extracted, controlled stimulation of these stem cells to form osteoblasts (bone forming cells) and odontoblasts (tooth forming cells) is relatively straightforward in these ‘well-behaved’ stem cells, and these cells can then be used in restorative dentistry for example. Stimulation involves biochemical factors which control differentiation pathways (‘morphogens’). In the case of dental pulp stem cells, mechanical factors such as fluid flow and hydrostatic pressure, also affect their behaviour and differentiation. Interaction with biocompatible scaffolds, used to support cells and shape new tissues, also affect dental pulp stem cell behaviour.
Despite their tendency towards osteogenic/odontogenic cell formation, dental stem cells are also able to differentiate into other cell types, including fat, neural, liver and many other cell types. This means their potential for medicinal use is not restricted to oral treatments. For example, dental stem cells could be used for blood vessel formation in treatment of heart diseases (such as coronary heart disease, where reduced blood-flow to the heart can eventually lead to an infarction (heart attack)), or for functional neural cell generation (for example in the treatment of Parkinson’s disease). Closer to their origin, dental stem cells are also investigated for treatment of periodontal (gum) disease through gingival regeneration. Each of the different dental stem cell lines are associated with slightly different cell types they are able to differentiate into, providing slightly different functions in the mouth as well as different wider potential for other treatment.
There are numerous further stem cell sources and types, not just mesenchymal or mesenchymal-like stem cells. Embryonic stem cells are pluripotent so could have great potential in medicine, but research has been limited by ethical concerns. Use of the wide variety of adult stem cells (Figure 1) has fewer restrictions. Adult stem cell transplants are already used in the treatment of some diseases (such as haematological malignancies or neuroblastoma). Haematopoietic stem cell transplants (from adult bone marrow or umbilical cord blood) are a well-established and essential treatment for some forms of leukaemia, helping to replace bone marrow and restore the immune system. Injuries or diseases in bone, eye and skin are often treated by grafts or implants, which need to contain (natural) stem cells for effective healing.
Otherwise current clinical application of stem cells is minimal. However, they are under investigation for use in many treatments, at a preclinical stage. Some of these investigations are discussed below in an oral and maxillofacial surgery context. Only time can tell what is going to emerge as established clinical interventions from this rich and active area of research.
Stem cells in tissue engineering
Tissue engineering involves the combination of cells, scaffolds and other biological materials with the goal of repairing or producing functional tissues and organs. Experimental treatments have included implantation of small arteries, skin grafts, cartilage and supplemental bladders, however these are yet to be used on a wider scale. Tissues from organs such as the heart, lungs and liver have been created in the laboratory, but are still far away from clinical applications. Potential pathways being investigated for tissue engineering include stem cells, 3D printing, gels and adhesives, and use of collagen scaffolds left after removing cells from a donor organ. As one would expect, it is an area in which stem cells (which can expand and differentiate into desired tissue) show great potential.
Repair of bone defects is a widely researched area, relevant across the body, including the head, neck and face. More maxillofacial-specific tissue engineering approaches include the retrieval of salivary gland function, cleft lip and palate repair, and the reconstruction of bone and skin defects. These various techniques and applications of stem cells are summarised in Figure 3. It should be noted that this is not an exhaustive list of all the many uses stem cells could have in this area.
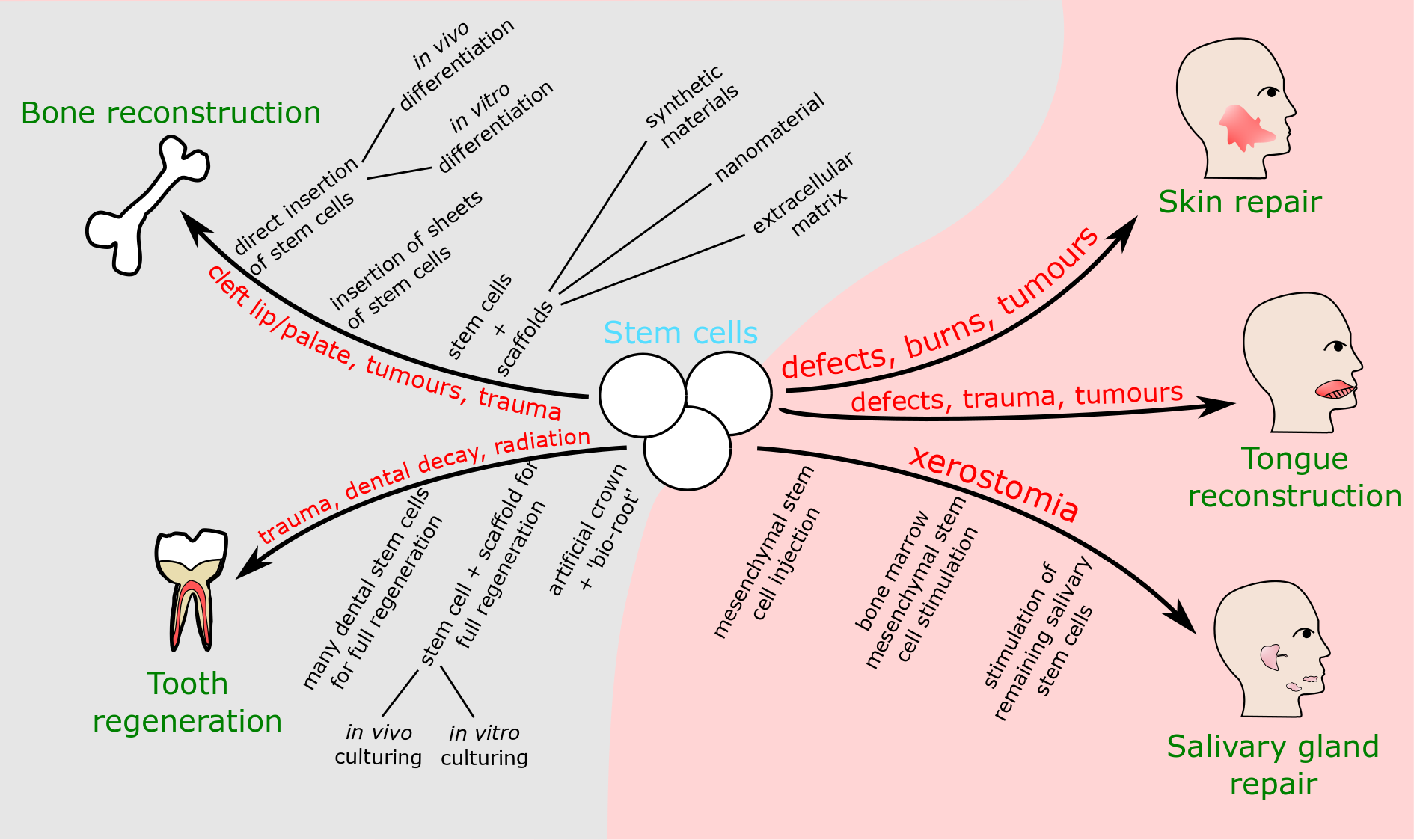
Reconstruction of hard tissue / bone defects
Reconstruction of bone defects is an important aspect of maxillofacial reconstructive surgery, for example in tumour surgery or in trauma surgery and several other interventions where large bone defects occur. Current reconstructive methods involve autologous bone (and soft tissue) grafts and free flaps. Whilst these are typically very successful in restoring a good degree of normal function and appearance, these methods are highly invasive and are invariably associated with some donor- and recipient-site morbidities. Stem cells have the potential to improve bone reconstructive surgery, restoring both bone structure and function. Mesenchymal stem cells are involved in the body’s own reconstructive procedures. They are able to differentiate into bone cells, or less directly induce repair mechanisms through production of growth factors (biochemical messenger molecules) which stimulate proliferation, differentiation and attraction of other cells. However, aging has a detrimental effect on natural mesenchymal stem cell function, potentially due to changes in bone marrow or mesenchymal stem cell interactions with other cells and the extracellular matrix. Therefore, improvements in the body’s own repair mechanisms or having to rely less on those, is a particular concern in the surgical treatment of elderly people.
There is a wide array of options being investigated for the use of stem cells in bone reconstruction (Figure 3). Stem cells can be
- injected directly;
- transplanted on scaffolds (scaffolds carry cells, fill space and act as a template for bone regeneration);
- attracted from other sites within the host (mediated by insertion of specific cytokines (specialised messenger proteins) which recruit the stem cells to sites of injury and help them proliferate and differentiate).
Whichever approach is taken, numerous decisions must be made within that approach for the most efficient repair process to be selected. If injecting directly, there is a choice between injecting the stem cells immediately and allowing them to differentiate in the host (in vivo), or manipulating the stem cells outside of the host (in vitro) to express specific genes or differentiate into the desired cell type. If using a scaffold, there are many different types and subtypes of scaffolds to choose between: synthetic scaffolds (calcium phosphate ceramics, metallic, polymeric); nanomaterial-based scaffolds (nanopatterned, nanofibrous or nanoparticular); extracellular matrix-based scaffolds (decellularised native extracellular matrix, decellularised engineered extracellular matrix, demineralised bone matrix). The examples here merely illustrate the complexity of options available for research and the extensive needs for testing (Figure 3) before any of these laboratory procedures will be fit for clinical practice. The goal for whichever approach taken is to restore bone structure and function. Functional restoration not only involves restoring the supportive function of bone for soft tissues, but also requires blood supply to provide bones with oxygen and nutrients, and to remove waste products. Therefore, an additional factor in the choice of approach is deciding which will allow sufficient regeneration of blood vessels. Addition of growth factors could help regrow blood vessels, while co-culturing with human umbilical vein endothelial cells, or the use of nanomaterials, have also shown improvements in this regard. Use of growth factors can lead to its own problems; malignancies could be triggered to develop or return. With bone repair common in oncological surgery, this is an obvious risk.
Cleft lip and palate is one of the most common birth defects and may require staged repair interventions, including bone, over many years into adulthood and aligned with body growth. Current treatment methods involve use of bone grafts, usually from autologous bone, which has some limitations and side effects. Use of stem cells could provide a more effective treatment. Recent studies have suggested that using sheets of stem cells lined up could be more effective than transplants of individual cells (whether alone or on a scaffold). This is based on the cells in sheets appearing to have more regular cell behaviour, such as in their interactions with other cells and the extracellular matrix, and in the number of molecules present on their surface. The sheets can also be layered to help form more complex and functional tissues.
The effectiveness of cell sheets was tested on mice, in which palatal bone was successfully regenerated through combination of layers of two types of stem cells (mesenchymal stem cells and stem cells from human exfoliated deciduous teeth). Both these cell lines were shown to form osteoblasts and bone tissue, and hence have potential for use in palate repair. This is an example of use of an animal ‘model’ in research. Animal models are used to imitate human conditions, for example due to similar responses to disease, similar genetics or similar anatomy. Mice are a particularly good model as they surprisingly have some very similar genetics and behaviour to humans, allowing good imitation of many human conditions. There are, of course, ethical concerns over use of animals in this way, and models have limitations, as they often do not show the same response as humans. However, these models are very useful in initial testing of treatments, reducing the chance of unexpected adverse effects on humans, and also useful in testing theories and whether novel avenues of treatment are worth pursuing or need modification. One could argue that a reasonably restrained and responsible use of animal models is an ethical obligation in some instances. For example, imagine trying to set up a randomised clinical trial of such palatal bone repair in 3 to 6 month old babies. Another example would be in utero cleft repair, while postulated to be a scar-less intervention, never took off because of the ethics involved and the risk of spontaneous abortion. Most of the experiments described below use animal models.
Replacement of missing teeth may also benefit from stem cell-based methods. Some animal studies (on dogs and pigs) have attempted to form full teeth through the use of single stem cells and scaffolds in an autografting process. Direct implantation of stem cell and scaffold allowed only dentine regeneration. Replicating and culturing the cells onto the scaffold while outside the body was more successful; this facilitated regrowth of dentine, roots and periodontium on implantation. Despite this success, there is an overall difficulty of regenerating full-sized teeth with roots and normal function. For complete tooth regeneration, research suggests that a combination of many different dental stem cell types would be required. An alternative approach is to use just one or two of these stem cell types to develop ‘bio-roots’ (replacement roots of teeth, a biological alternative to dental implants), onto which prosthetic crowns can be fixed. Stem cells from the apical papilla and the periodontal ligament have been used in pig jaws, with some short-term success so far. A variety of non-dental stem cells have also been trialled and shown some success in tooth development, including bone marrow mesenchymal stem cells and, interestingly, human urine-derived induced pluripotent stem cells. The urine derived induced pluripotent stem cells were used to form sheets of dental epithelial cells, which eventually differentiate into enamel. This was combined with mesenchymal stem cells from mice, which form dental pulp, cartilage and bone-like structures, in a novel system which formed tooth-like structures.
Reconstruction of soft tissue defects
Salivary gland repair to restore saliva production is an area in which stem cells show potential. There are two severe xerostomia (dry mouth) conditions: radiation-induced xerostomia from radiotherapy applied to the head and neck region, and Sjögren’s syndrome, an autoimmune condition. Both conditions destroy the acinar (saliva-producing) cells in the salivary glands. Current treatments focus on alleviating symptoms, but fail to effectively restore function and/or quality of life.
In salivary gland repair, mesenchymal stem cells are again the main cell type being investigated for use in treatment. The theory is that they could be injected, before proliferating and differentiating, to form enough new acinar cells to secrete a functional amount of saliva. Mouse models (see above) for both conditions have shown significantly increased salivary secretion following injection of mesenchymal stem cells. For Sjögren’s syndrome, small initial safety trials on humans displayed no harmful effects and doubled original salivary flow just a month after treatment.
Mesenchymal stem cells do not necessarily need to be injected for treatment. Mesenchymal stem cells from the host bone marrow can be stimulated to move to the salivary glands and help regenerate tissue, through releasing growth and survival factors which could help remaining salivary gland stem cells survive, proliferate and differentiate into new acinar cells. Mesenchymal stem cells are also not the only type of stem cells that could be used. For example, salivary gland stem cells could be removed before radiotherapy and reimplanted after radiation treatment. There they would hopefully differentiate back into functional salivary gland cells and a functional salivary gland. In cases where radiation exposure is less intense, finding ways to stimulate remaining salivary gland stem cells could allow them to proliferate and differentiate to restore function. Overall, stem cells either from the salivary glands or elsewhere could be used to produce new acinar cells, restoring and promoting saliva production – with the obvious exception of salivary gland malignancies.
Repair of skin defects is another branch of soft tissue reconstruction in which stem cells could prove useful. Stem cells naturally residing in skin are able to repair small wounds, but are unable to fix larger wounds which would become chronic without treatment. Current treatment involves the use of skin grafts and flaps for the repair of defects. Stem cells could provide an improved treatment. As with other areas of tissue engineering, their abilities to self-renew and differentiate into different tissues are key. Here the reformed tissues could include not only skin but blood vessels and skin appendages such as hair follicles, sweat and sebaceous glands, which would improve the aesthetics and function after reconstruction. The secretome of stem cells (the proteins expressed and released by the cells) gives them further potential:
- release of factors which supress the immune system slightly, helping to prevent excessive inflammation;
- release of factors which promote keratinocyte (most common type of skin cell) migration should help skin healing;
- release of growth factors promoting blood vessel formation can help improve healing and/or establishing grafts and flaps.
Bone marrow mesenchymal stem cells and adipose-derived stem cells have shown all these promising characteristics. These and other stem cell types have been tested and shown regenerative capabilities for skin repair in animal models, especially rats. However, problems have been encountered in effective application of stem cells to burn wounds, and there have been very few human trials. Two recorded trials were on individual patients with 40 to 60 % of their bodies burned, including burns going through the full thickness of skin. Both trials used donor bone marrow mesenchymal stem cells cultured into a single layer before application, with one trial directly adding the layer on top of burns, and one spraying it on. Each approach caused improved vascularisation and granulation (formation of new connective tissue and capillaries), allowing successful use of skin grafts later on, which were unsuccessful without prior stem cell treatment. Currently, stem cells are far from regular use in skin replacement, but have shown potential for improving effectiveness of regular treatment and have potential to replace it in the future.
Repair of muscle defects , in particular tongue reconstruction, is another area in which stem cells could be used in oral and maxillofacial surgery. The tongue is vitally important for multiple functions such as speech, swallowing and breathing, so effective treatment needs to restore sufficient functionality. Current reconstructive surgery after partial or complete resection of the tongue uses a range of free flaps. Recent developments have included the use of a decalcified collagen scaffold to replace large gaps in tongue tissue.
Stem cells could both improve on current treatments and provide novel therapeutic methods. The recent use of collagen scaffolds could potentially be improved by the addition of stem cells (or myoblasts, muscle forming cells) from the remaining tongue tissue to the scaffold, improving regeneration of muscle and other cells in the tongue. Studies on rat models have shown some promise, for example demonstrating improved muscle quality in stem-cell regenerated tongue tissue, and effective healing of tongue scarring by bone marrow mesenchymal stem cells. The latter could be very useful as scarring at the bottom of tongue / floor of the mouth can cause severe dysphagia (difficulty swallowing). Mesenchymal stem cells have shown promise in the repair of muscular defects elsewhere in the body (in animal models). For example, there have been reports about restoring function in rodent heart attack models, and repairing urethral muscle defects in canines to restore the ability to urinate. Overall, stem cells have shown some early promise in repairing muscular defects in animal models, including in the tongue. However, these laboratory investigations are yet to reach human trials to fully assess their future therapeutic value.
Stem cell involvement in disease
Graft versus host disease
Graft versus host disease affects nearly 50 percent of people who received a donor stem cell transplant (an allograft), typically for the treatment of haematological malignancies. It is a leading cause of disease and death, aside from relapse, after stem cell transplants. Transplanted T cells (an essential type of immune cell, part of the immune system) are thought to be the key cause of this condition. T cells from the donor recognise healthy cells of the recipient as foreign, a threat which activates these cytotoxic (cell destroying) T cells, which then kill the healthy host cells. There is a wide variety of responses to T cells however, so it is thought that other cells are involved, including B cells. B cells are another type of immune cell, which normally produce antibodies to inhibit pathogens and their toxins. An example of their action in graft versus host disease is in gender-mismatched transplants. When females donate to males, their antibodies bind male-specific antigens, causing damage to healthy cells. Loss of mechanisms to control immune reaction to self-tissues worsens the condition. This is thought to occur due to the overproduction of cytokines such as B cell-activating factor (causing lots of activated circulating B cells), and damage to the epithelium in the thymus (where T cells are made). This causes loss of regulation and selection of T and B cells, hence allowing them to attack host cells. Regulatory T cells, which usually supress the immune system, may also be affected. The frequency and severity of graft versus host disease displays clearly the risks involved in all stem cell treatment schemes. It is important to control stem cell differentiation and avoid any unnecessary immune cell production.
The cancer stem cell model
Another example of stem cells causing damage is in their proposed role in the development of malignancies. This consideration is not about a particularly aggressive, specific type of a general ‘cancer stem cell’. Rather, the term cancer stem cell refers to one of two main models aiming to explain in detail the development and maintenance of malignancies: the stochastic model, and the cancer stem cell model. The stochastic model is based on the assumption that each malignant cell has the ability to divide, hence allowing maintenance and growth of malignancies.
However, there is growing evidence for the cancer stem cell model, where only a subpopulation of malignant cells has stem cell-like properties such as the ability to self-renew, and to differentiate into the new cells for maintenance and growth of a malignancy (similar to the cellular variety in healthy tissues). The cancer stem cell model makes no limiting assumptions about the abundance of the cancer stem cell subpopulation or its properties. It is a generalised model, and the stochastic model can actually be thought of as one of the extremes of the cancer stem cell model (where all malignant cells happen to be cancer stem cells).
Since malignant cell lines are instable and evolve, continually producing new mutations, the cancer stem cell model is particularly well suited to explain the evolution of heterogeneity in malignant tumour tissue: new lines of cancer stem cells as well as non-renewing (differentiated) malignant cells, all with a range of properties, can emerge from the initial cancer stem cell pool. The model thus suggests that cancer stem cell subpopulations can provide mechanism for resistance to cancer treatments, facilitating both regression (spontaneous remission of malignancies), recurrence, and progression of disease to metastasis. Here we discuss the implications of the cancer stem cell model on the key stages of development of malignancies: development (initiation), growth (promotion), and spreading (metastasis) (Figure 4).
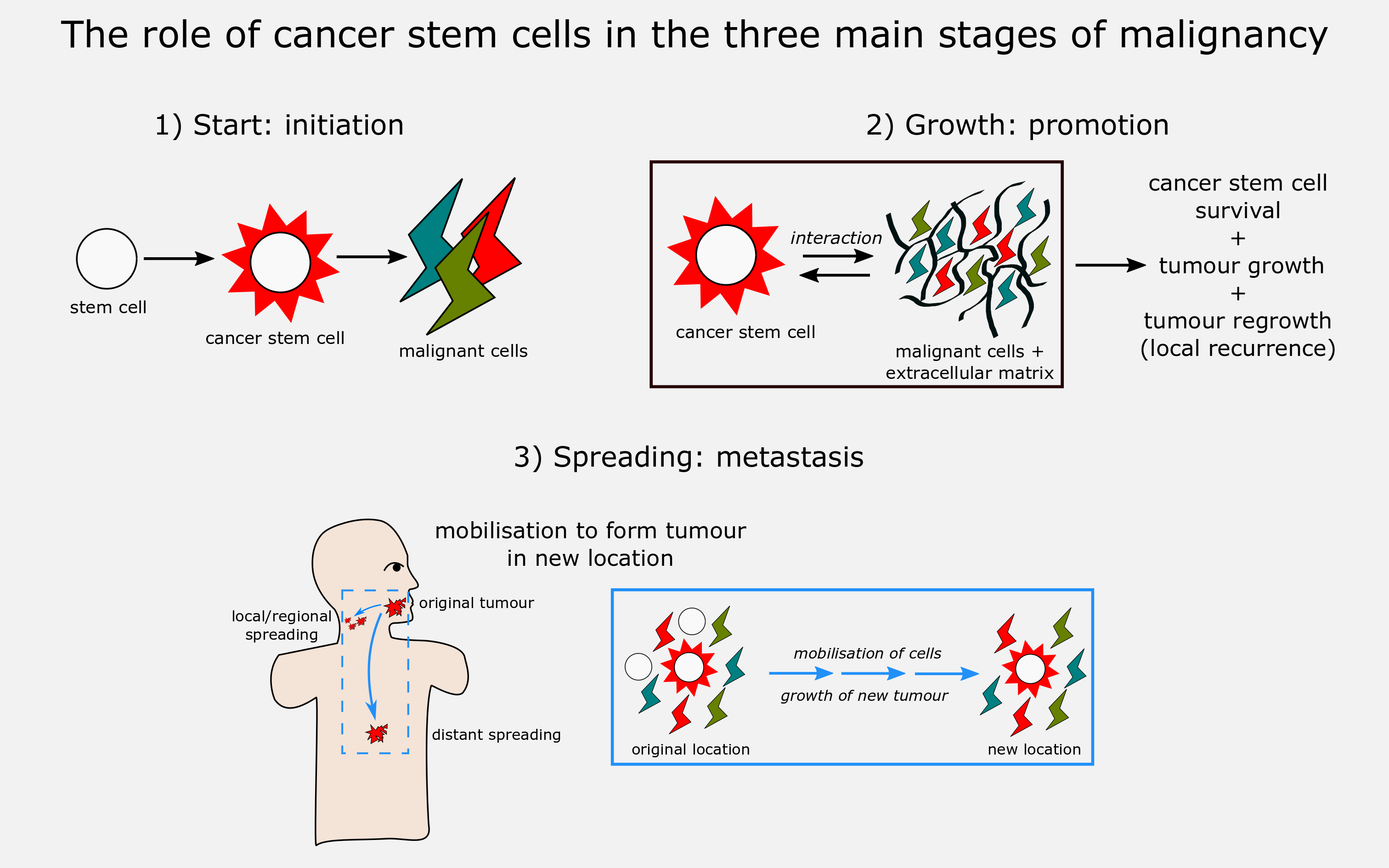
Having a valid model to describe and understand carcinogenesis is vitally important for the rational development of efficient treatment schemes for all kinds of malignancies. If the situation is correctly reflected by the stochastic model, this implies that any curative treatment of a malignancy requires the elimination of every single cell of a malignancy. In contrast, the stem cell model suggests that curative treatment, or at least long-term remission, can be achieved by selectively targeting the cancer stem subpopulation(s) that is/are responsible for the growth and maintenance of a particular malignancy. This, in turn, implies that treatment planning needs to be able to correctly & safely identify these cell lines, and that there have to be specific agents that will reliably target and selectively eliminate these cancer stem cell subpopulations. This entire, broad theme is the subject of intense research all over the world, and recent dramatic methodology advances in genetics are at the core of many investigations.
Initiation is the stage in which cells transition from healthy to malignant cells. Although cancer stem cell origin is unclear thus far, one prominent theory is that a change of conditions can induce regular stem cells residing in tissue to transition into cancer stem cells (the cancer stem cell model as such does not directly imply a functional or developmental relationship with normal stem cells; it just assumes the presence of (a) subpopulation(s) of malignant cells). For example, a high concentration of reactive species (present during inflammation, or continuous exposure to noxious chemicals such as components in cigarette smoke or general air pollution) can cause DNA mutations. These mutations could cause normal stem cells to transition into cancer stem cells, which would then allow tumour growth through their proliferation and differentiation into tumour cells.
Promotion is the stage in which malignancies survive and grow at the primary tumour site. As well as their obvious role in growth, a key part of cancer stem cell involvement here is in their interaction with the tumour microenvironment, including other tumour cells and the extracellular matrix. The microenvironment influences cancer stem cell populations, and cancer stem cells influence the microenvironment (as is sometimes stated: a malignancy and its host evolve together). There is an important common effect in these mutual bidirectional interactions: the support of cancer stem cell survival and tumour growth. Cytokines (specialised messenger proteins) are a key component of these interactions. For example, cancer-associated fibroblasts (part of the microenvironment) secrete cytokines which support cancer stem cell survival. In the other direction, cancer stem cells release cytokines (and chemokines) to cause inflammation, attracting tumour-associated immune cells into the microenvironment, which in turn promote tumour proliferation.
Two specific areas in which the microenvironment - stem cell interactions are important, are in hypoxia and drug resistance. Hypoxia (lack of oxygen) provides an example of the effect of changing microenvironment conditions, and of bidirectional signalling. It seems that hypoxia regulates cancer stem cell properties and can even promote transition of normal stem cells to cancer stem cells. However, tumours need oxygen to survive and grow, and hence cancer stem cells in order to maintain further tumour growth, promote angiogenesis (growth of blood vessels). In turn, these new endothelial cells signal back to promote cancer stem cell expansion. None of these mutual functional relationships are simple, or single-cause interrelations. Hypoxia clearly is an unfavourable condition for malignant cell lines to survive and one could rightly conclude that starving malignant cell lines of oxygen will eventually kill them. However, in order for high-energy photon radiation to be effective at destroying malignancies, it is important that the tumour environment is not hypoxic – the mechanism by which radiotherapy destroys cells is strongly dependent on the presence of oxygen.
Cancer stem cells could also play a key role in developing drug resistance, in a number of ways. Through resistance to DNA damage, altering of drug metabolism, upregulation of drug efflux (pumping the drug out of the cell), and survival-promoting epigenetic changes (changes to gene regulation), cancer stem cells can provide resistant populations which survive chemotherapy and other treatments, such as immunotherapies. The mechanisms at work are similar to those in the development of resistance of bacterial cell lines to antibacterial agents and in both cases lead to enhanced selection and growth of resistant cell lines. These mechanisms further support recurrence and metastasis of malignancies. There is also a theory that cancer stem cells can induce non-cancer stem cells to temporarily gain resistance properties and survive treatment.
Resistance of cancer stem cells to treatment is a key property which gives malignancies the potential to survive treatment and re-establish themselves (recurrence) and/or spread (metastasis). The interaction of cancer stem cells with their environment is also vital here, the interactions allowing tumour growth and angiogenesis at a new (regional or distant), site to form a ‘pre-metastatic niche’. This essentially prepares favourable conditions for the establishment of a new colony of malignant cells to settle and grow in a new location. Inflammation triggered by cancer stem cells (see above) can also promote metastasis.
In summary, current systemic treatments (chemotherapy and immunotherapy) for malignancies can be challenged by the resistance properties innate to, or acquired by, ‘cancer stem cells’.